Figure 21 shows the distribution of coal, oil and gas fields of the Gippsland Basin. Multiple resources are viable for development in the Gippsland Basin including groundwater use, hydrocarbon extraction, coal mining and geological storage of carbon dioxide (CO2) (Varma and Michael, 2012). A summary of coal, hydrocarbon and potential CO2 reservoir resources is provided herein. Groundwater is discussed in detail in Section 1.1.4.
Figure 21 Distribution of coal fields and resources, oil and gas fields of the Gippsland Basin
Data: Geoscience Australia (Dataset 2), Department of State Development, Business and Innovation (Dataset 3), Department of Environment and Primary Industries (Dataset 4) Department of State Development, Business and Innovation (Dataset 5)
An overview of coal resources is provided here. More detailed discussion of coal resources in the Gippsland Basin bioregion will be presented in companion product 1.2 of the Bioregional Assessment (Coal and coal seam gas resource assessment).
Black coal
Black coal occurs within the Cretaceous Strzelecki Group of the Gippsland Basin. The coals are mainly high-volatile bituminous with 5 to 10% moisture, 30 to 35% volatile matter and 6 to 12% ash. Vitrinite reflectance is up to 0.9% Rv for buried strata, and 0.5 to 0.67% Rv for strata in outcrop (Holdgate, 2003). Some black coal production has occurred historically within the Gippsland Basin where local uplift and erosion stripped overburden. This has mainly been in the vicinity of the Narracan Block, where black coal was mined from Lower Cretaceous Strzelecki Group rocks, most notably at Wonthaggi. Mining of black coal occurred between 1864 and 1970 and production amounted to approximately 23 million tonnes (Mt) (Holdgate, 2003).
Brown coal
Brown coal deposits formed in the Gippsland Basin during the Cenozoic and are hosted within the Latrobe Group and Latrobe Valley Group. The brown coal deposits of the Latrobe Valley Depression are the largest of their type in the world (Holdgate, 2003). Currently, large scale open-cut mining occurs in the Latrobe Valley at a rate of approximately 40 Mt per year from three mines at Hazelwood, Loy Yang and Yallourn. This production supplies most of Victoria’s electricity needs. Historically, small-scale brown coal mining occurred at Gelliondale (Alberton Depression) (Holdgate, 2003). Holdgate (2003) described the size of the brown coal resource of the Latrobe Valley as effectively without limit, stating:
Whether any further future developments occur depends very much on satisfying greenhouse gas emission standards, because there are currently no limits to the economically winnable reserves, at least for many hundreds of years to come.
A detailed coal resource inventory for all of Victoria including the Gippsland Basin was undertaken in 2007 (GHD, 2007) providing an estimate of potential economic coal resources. Table 12 summarises the distribution of known brown coal resources in the Gippsland Basin.
Table 12 Brown coal fields of the Gippsland Basin
Data: GHD (2007)
Note there are no coal fields in the Lakes Entrance Platform or the Lake Wellington Depression, however, thin coal seams are intercepted under deep cover in boreholes within these regions)
The Traralgon Formation contains the largest brown coal deposits in the Gippsland Basin (Holdgate et al., 2000). Two coal seams are named within the Traralgon Formation, the T1 and T2 seams. Seam thicknesses often exceed 100 m. Where the seams occur in stratigraphic superposition, they form a total thickness of over 150 m.
The younger T1 seam is recognised in the Latrobe Valley Depression, north and west of the Rosedale Monocline/Fault, extending a short distance to the west of Loy Yang where the westerly limit of the T1 coal seam is controlled by the Morwell Monocline/Fault (Holdgate et al., 2000). It is present across the Baragwanath Anticline and in the Lake Wellington, Seaspray and Alberton depressions (Holdgate et al., 2000). The northern limit of the T2 coal seam is controlled by the Rosedale Monocline/Fault. It is present on the Baragwanath Anticline, and in the Lake Wellington and Seaspray depressions (Holdgate et al., 2000). T2 coals can also extend over 25 km offshore (Holdgate et al., 2000).
Traralgon Formation coal seams near the basin margins have moisture contents around 55%. With deeper burial and folding, moisture content decreases and moistures below 50% are normally found (Holdgate et al., 2000). On average, there is a decrease of 2.7% in moisture content between the T1 and T2 seams (Holdgate et al., 2000). The coals have low ash content (mean 2.9% db). Samples collected from more deeply buried coals are generally higher in rank than those collected at more shallow depths (Holdgate et al., 2000).
The Traralgon Formation coal seams subcrop generally beneath < 30.5 m overburden across the Baragwanath Anticline uplift area where, out of an indicated resource of 345 billion tonnes (Bt) across the basin, approximately 10 Bt are considered economically recoverable reserves (Holdgate et al., 2000). Elsewhere in the basin, the Traralgon coal seams are deeply buried, for example in the Seaspray Depression they are covered by 300 to 700 m thick Seaspray Group limestones. They are unlikely to be mined in the areas of thick cover, but may constitute a possible coal seam gas resource (Holdgate, 2003).
The Morwell and Yallourn Formations of the Latrobe Valley Group host the most economic deposits of brown coal (Holdgate et al., 1995). They are located in the Latrobe Valley Depression. The Latrobe Valley Group can be up to 700 m thick, of which more than two-thirds can be brown coal. The thickness of individual seams, five of which are greater than 100 m thick, and the vertical multi-seam levels are without parallel in the world (Holdgate et al., 1995). In some areas, superposition of seams results in coal thickness in excess of 200 m, for example on the western flank of the Loy Yang Dome, the Morwell 1A, 1B and 2 seams all combine, producing up to 230 m of continuous coal (Holdgate, 2003). Around 30 Bt of economically recoverable reserves have been identified along structural highs within the Latrobe Valley Depression (Holdgate et al., 1995).
Moisture contents of the Yallourn coals range from 64.4% to 68.1% (Holdgate, 2003). Moisture contents of Morwell coals range from 54.4% to 64.6% (Holdgate, 2003). Moisture contents generally decrease with coal age and depth of burial. For any given depth, moisture content is generally higher on anticlines due to lowered intensity of compression than in adjacent synclines (Holdgate et al., 2007). Ash contents are low, ranging from 1.8% db to 3.2% db in the Yallourn coals and 1.5% db to 3.8% db in the Morwell coals (Holdgate, 2003) where ash contents are reported on a dry weight basis (db).
The Morwell 2 sequence comprises three main subseams, the M2A, M2B and M2C. In the north-western area of the Latrobe Valley, the three seams combine to form over 150 m of continuous coal (Holdgate et al., 1995). The sequence thins eastward in the down-dip directions. The Morwell 1B sequence (M1B) comprises 120 m of continuous coal (Holdgate et al., 1995). The Morwell 1A (M1A) sequence has a similar thickness to the M1B (Holdgate et al., 1995). At Loy Yang and Morwell, the M1A and M1B seams join to form over 180 m of continuous coal, while elsewhere the seams are separated by a thin marine clay. The M1A coal can be up to 100 m thick in the main coal fields, but over large areas of the central Latrobe Valley the seam splits into interbedded clay, coals and lesser sands (Holdgate et al., 1995).
The Alberton coal measures are an age equivalent of the Morwell Formation in South Gippsland. The coal seams within the Alberton coal measures are the upper A seam (55 m thick) and the lower B seam (15 m thick). They average 60% moisture content (Holdgate et al., 2007).
The Yallourn sequence comprises up to 110 m of coal in areas near the western end of the Latrobe Valley. The seam spatially overlies the Morwell 2 seam. The major Yallourn depocentre was on the downthrown east side of the Morwell Monocline (Holdgate et al., 2007). On the upthrown west side, the Yallourn sequence is truncated by Pliocene erosion. West of the Yallourn Monocline, the seam is eroded off the uplifted Haunted Hill Block. An age equivalent, the Moe Seam occurs in the Moe Swamp sub-basin, west of the Haunted Hills Block with a thickness of 40 m (Holdgate et al., 2007).
1.1.3.4.1 Geological hazards
Subsidence
Lowered artesian pressures in the aquifers of the Latrobe Valley resulting from coal mine depressurisation has caused increased effective stress within the sediments of the Latrobe Valley Group, with resulting consolidation and subsidence. These are variable due to complexities in the aquifers, aquitards and overlying sedimentary deposits (Neilson et al., 2003).
Although subsidence has not been detected along the coast of the Gippsland Basin (Department of Environment and Primary Industries, 2014; Sjerp and Charteris, 2008), predictive subsidence modelling suggests that subsidence may occur along the coast as a result of the lowering of hydraulic heads in the Latrobe Group Aquifer, caused by offshore oil and gas production, onshore groundwater extraction for irrigation and coal mine dewatering (Freij-Ayoub et al., 2007). Based on their projections of future groundwater level in the Latrobe Group Aquifer, Freij-Ayoub et al. (2007) predicted maximum coastal subsidence of between 480 and 1208 mm in the year 2056.
1.1.3.4.2 Conventional and unconventional hydrocarbons
Conventional oil and gas
The Gippsland Basin is one of Australia’s major crude oil and natural gas provinces. More than 400 petroleum exploration wells have been drilled in the basin and approximately 90,000 line km of two-dimensional seismic data and more than forty three-dimensional seismic surveys have been acquired. Consequently, exploration within the basin is mature in comparison with other Australian basins, particularly in the Central Deep region of the basin. Outside this region however, the basin is relatively under-explored in comparison with other prolific hydrocarbon producing basins around the world (Geoscience Australia, 2014).
Most demonstrated oil and gas resources are in the offshore portion of the basin. Oil and gas are mainly produced from structural and structural/stratigraphic traps within the Oligocene, Eocene, Paleocene and Late Cretaceous marine, marginal marine and continental clastic sequences. Liquid hydrocarbon production peaked in 1985 and has gradually declined since then. Table 13 provides an overview of produced and remaining conventional oil and gas demonstrated resources in the Gippsland Basin. There is an expectation that further recoverable conventional gas resources will be discovered in the Gippsland Basin (Bradshaw et al., 2012; Pollastro et al., 2012).
Table 13 Gippsland Basin oil and gas resources in units of Petajoules (PJ)
Produced (PJ) |
Remaining (PJ) |
|
---|---|---|
Crude oil |
25 536 |
1699 |
Condensate |
753 |
|
LPG |
646 |
|
Conventional gasa |
8791 |
9300 |
Data: Geoscience Australia (2010), aBradshaw et al. (2012)
The main period of hydrocarbon generation and expulsion is thought to have commenced in the Miocene as a result of increased sedimentary loading of the Cenozoic carbonate sequences. In the major depocentres of the basin, restricted areas underwent an earlier phase of generation and migration around the middle Eocene. At that time, no regional Lakes Entrance seal was in place and any traps would have involved older intra-Latrobe Group sealing units and earlier formed traps (Geoscience Australia, 2014).
Onshore, three sparsely explored hydrocarbon play fairways have been identified: the Early Neocomian part of the Strzelecki Group, the Golden Beach Subgroup, and the intra-Latrobe Group at target depths ranging from 800 to 4000 m (Chiupka, 1996). Table 14 is an overview of the Gippsland Basin petroleum system.
Unconventional hydrocarbons
At present, the Victorian unconventional gas industry is at a very early stage. It is not yet known whether coal seam gas extraction is economically viable in the Gippsland Basin. A moratorium on hydraulic fracturing has been in place in Victoria since August 2012 (Ross and Darby, 2013). The moratorium has slowed commercial exploration for unconventional gas resources.
Harrison et al. (2012) divided the onshore Gippsland Basin into two distinct zones that have different prospectivity for unconventional gas resources: the ‘Western Block’ where the lower rift sequence of the Cretaceous Strzelecki Group is widely exposed due to multiple episodes of basin inversion along north-easterly trending faults linked to the basement, and the ‘Eastern Depression’ where the Strzelecki Group is more simply distributed across the basement, with structure still dominated by the original east-west extensional faults.
Table 14 Gippsland Basin petroleum system
Data: Geoscience Australia (2015)
Coal seam gas
Harrison et al. (2012) noted that groundwater flowing off the highlands and Strzelecki Ranges toward the petroleum production areas could be continually bringing new nutrients into Traralgon Formation coal sequences and removing wastes to enable microbial production of methane. Temperature ranges in the Traralgon Formation are close to or overlapping with the survival threshold for micro-organisms, allowing a small possibility of microbial methane production (Harrison et al., 2012). Further discussion of coal seam gas development potential will be provided in companion product 1.2 of the Bioregional Assessment (Coal and coal seam gas resource assessment).
Tight gas
The Strzelecki Group experienced much higher temperatures in the past (during the middle Cretaceous, 100 to 90 Ma). The Strzelecki Group may be considered the source, reservoir and seal for tight gas. Fluvial source rocks are carbon rich with numerous flecks of coal and plant debris clearly visible in core and outcrop samples, likely being gas-prone kerogen sources, and the required thicknesses of around 500 m of sediments occur throughout the basin except at its margins. Additionally, the modern thermal regime includes regions of the Strzelecki Group that appear to be in the gas generating window (150 °C). However, the previous higher temperatures may have already stimulated gas production in the past (Harrison et al., 2012). Harrison et al. (2012) also commented that there may be regions of the Strzelecki Group where current temperatures are higher than paleo-temperatures and might be in the gas generating window.
Bradshaw et al. (2012) reported that the Gippsland Basin contains approximately 1853 PJ of tight gas for the Wombat, Gangell and Triforn/North Seaspray reported discoveries. These discoveries are onshore, within the Strzelecki Group in the Seaspray Depression.
Shale gas
Bradshaw et al. (2012) did not identify the Gippsland Basin as being prospective for shale gas formations. There are no shales of deep marine origin with algal kerogen in the Gippsland Basin.
1.1.3.4.3 Other reservoir resources
Water
The sedimentary units of the Gippsland Basin also host significant groundwater resources that are utilised for a variety of industries. Groundwater will be discussed further in Section 1.1.4.
Geothermal
Harrison et al. (2012) included information on geothermal prospectivity in the Gippsland Basin, particularly onshore. Harrison et al. (2012) noted that Cenozoic sediments in the onshore Gippsland Basin have lower thermal conductivity on average than comparable rocks around the world and that this leads to higher temperatures in the subsurface that may enable low temperature geothermal energy use (e.g. supplementary water heating at coal fired power stations potentially increasing coal use efficiency). Groundwater temperatures ranging from 30° C to 70° C have been recorded from depths between 500 and 1000 m in the Gippsland Basin. The hottest groundwater is in the Latrobe Valley and Lake Wellington depressions. Geothermal gradients in these areas range from less than 2° C/100m to over 10° C/100m (Leonard and King, 1992).
CO2 sequestration
The Gippsland Basin has had a high level, preliminary assessment undertaken for geological storage reservoirs for CO2 sequestration. Goldie Divko et al. (2009) provided a summary of the geological carbon storage potential of the onshore Gippsland Basin identifying preliminary targets for storage as well as preliminary estimates of potential storage volumes, including within the Strzelecki Group and Latrobe Group. Goldie Divko et al. (2009) emphasised that significantly more work investigating fault permeability and seal integrity is required to more adequately assess CO2 storage potential. Offshore, the Gippsland Basin has been identified as a highly prospective area for CO2 sequestration (Geoscience Australia, 2014).
The principal reservoir target is the Latrobe Group and the potential storage areas are generally current and future depleted oil and gas fields, located in the northern and central parts of the offshore basin. This poses a reservoir use optimisation problem with future CO2 storage potential depending on oil and gas resource extraction scheduling. Areas of near-shore and southern parts of the basin have recently been made available for exploration for CO2 sequestration via the Offshore Greenhouse Gas Storage Acreage Release (Geoscience Australia, 2014).
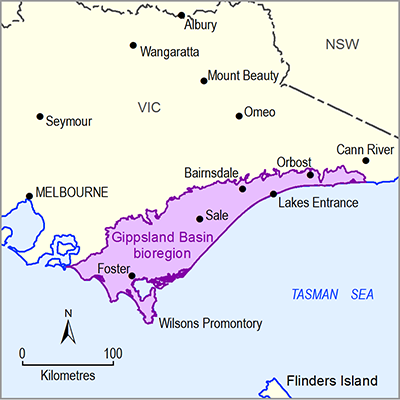