- Home
- Assessments
- Bioregional Assessment Program
- Clarence-Moreton bioregion
- 2.6.2 Groundwater numerical modelling for the Clarence-Moreton bioregion
- 2.6.2.8 Uncertainty analysis
- 2.6.2.8.2 Factors not included in formal uncertainty analysis
The major assumptions and model choices underpinning the Clarence-Moreton groundwater model are listed in Table 9. The goal of the table is to provide a non-technical audience with a systematic overview of the model assumptions, their justification and effect on predictions, as judged by the modelling team. This table is aimed to assist in an open and transparent review of the modelling.
In the table each assumption is scored on four attributes using three levels; ‘high’, ‘medium’ and ‘low’. Beneath the table, each of the assumptions are discussed in detail, including the rationale for the scoring.
The data column is the degree to which the question ‘if more or different data were available, would this assumption/choice still have been made?’ would be answered positively. A ‘low’ score means that the assumption is not influenced by data availability while a ‘high’ code would indicate that this choice would be revisited if more data were available. Closely related is the resources attribute. This column captures the extent to which resources available for the modelling, such as computing resources, personnel and time, influenced this assumption or model choice. Again, a ‘low’ score indicates the same assumption would have been made with unlimited resources, while a ‘high’ value indicates the assumption is driven by resource constraints. The third attribute deals with the technical and computational issues. ‘High’ is assigned to assumptions and model choices that are dominantly driven by computational or technical limitations of the model code. These include issues related to spatial and temporal resolution of the models. The final, and most important column, is the effect of the assumption or model choice on the predictions. This is a qualitative assessment by the modelling team of the extent to which a model choice will affect the model predictions, with ‘low’ indicating a minimal effect and ‘high’ a large effect. Especially for the assumptions with a large potential impact on the predictions, it will be discussed that the precautionary principle is applied; that is, the hydrological change is over rather than under estimated.
Table 9 Qualitative uncertainty analysis for the groundwater model of the Clarence-Moreton bioregion
CSG = coal seam gas
2.6.2.8.2.1 Single geological and conceptual model
The development of the groundwater model was based on a single conceptualisation underpinned by the geological model (companion product 2.1-2.2 (Raiber et al., 2016a) and companion product 2.3 (Raiber et al., 2016b) for the Clarence-Moreton bioregion). The uncertainties associated with the three-dimensional geological and conceptual hydrogeological model are highlighted in companion product 2.3 (Raiber et al., 2016b) and summarised briefly below.
Three-dimensional geological models are created from datasets that are inherently uncertain because they sample the subsurface at a limited resolution (Wellmann et al., 2010; Raiber et al., 2012; Raiber et al., 2016b). Uncertainties in three-dimensional geological models can arise from various factors with data density, data quality, geological complexity and conceptual uncertainties being the most common sources of uncertainties (e.g. Mann, 1993; Davis, 2002; Raiber et al., 2012; Raiber et al., 2016b).
In the three-dimensional geological models developed as part of the Assessment, geological uncertainty is mostly related to the lack of well-intersection data of deeper sedimentary bedrock formations. In addition, the lack of seismic data in some areas is another source of uncertainty. This means that the geological contacts between the volcanic and sedimentary bedrock units as well as those between the different sedimentary bedrock units are uncertain in some areas (Raiber et al., 2016b).
One particular area of high uncertainty in the geological model occurs near the crests of the Lamington Volcanics at the boundary between the Logan-Albert and Richmond river basins. However, this uncertainty is likely to have a very limited effect on the predictions due to the well-established dominant hydrological role of the Lamington Volcanics in this area. Furthermore, there are very few model nodes there. In contrast to this area of high uncertainty, the interfaces between the shallow alluvial and volcanic and sedimentary bedrock aquifers are comparatively well constrained in most parts of the groundwater model domain due to an abundance of groundwater bores with lithological and/or stratigraphic data.
Importantly, although there are many geological uncertainties across the entire Clarence-Moreton bioregion, the confidence level in aquifer geometry and the presence and continuity of aquitards is highest in the additional coal resource development area.
The existing groundwater monitoring network likely explains only a small component of the hydrodynamics of the Clarence-Moreton Basin. Based on the existing data, groundwater dynamics in shallow alluvial aquifers are relatively well understood. However, the lack of nested groundwater-monitoring bores means that it is very difficult to identify vertical groundwater fluxes between different hydrostratigraphic units within the Richmond river basin (Raiber et al., 2016b). Note that auxiliary evidence from hydrochemical data (i.e. the freshness of most alluvial groundwaters at the eastern boundary of the Clarence-Moreton Basin) within the Richmond river basin and other catchments within the Clarence-Moreton bioregion (Section 2.3.2.2.4 in companion product 2.3 for the Clarence-Moreton bioregion (Raiber et al., 2016b)) suggests that discharge from sedimentary bedrock is not the major source of water for alluvial aquifers.
The geological and conceptual models are limited by data availability, in terms of their density, quality and type. This is particularly relevant to the hydraulic properties of aquitards, where currently only very limited data are available. Any additional information would reduce the uncertainty in the geological model and thus has the potential to change the conceptualisation of the groundwater system. The data column in Table 9 is hence scored ‘high’.
It is technically possible to formalise the development of geological models and conceptual models to stochastically account for the sources of uncertainty in the groundwater model that they underpin, for example, see Rojas et al. (2008). The discretisation of a geological model into a numerical MODFLOW model grid is almost always associated with a loss of information and the potential introduction of artefacts due to the idiosyncrasies of the MODFLOW code that requires spatially continuous model layers. In the Clarence-Moreton case, geological layers had to be converted to spatially continuous layers; a process that involved multiple steps of splitting the three-dimensional geological model layers, adding thin continuous dummy layers outside the original extent of the layers followed by re-assembly. While a recent version of MODFLOW, namely MODFLOW-USG, can accommodate spatially discontinuous layers, it was not available at the start of the modelling task. The technical aspect is therefore scored ‘medium’ in Table 9. The operational constraints in BA however prevent going along those alternative pathways, mostly due to the large development time required to formalise and script the development of geological models and the considerable computational load required to jointly run the geological and groundwater model. The resources and technical attributes are assigned a ‘medium’ score accordingly.
This limitation will be addressed as part of a CSIRO strategic project ‘Next generation methods and capability for multi-scale cumulative impact assessment and management’ (sub-theme ‘Tracer-based improvement of groundwater model conceptualisation and predictability’).
Notwithstanding the ‘high’ score for data and ‘medium’ score for resources, the overall impact on predictions is scored ‘medium’. The most basic conceptualisation of the system, relevant to the impact assessment of CSG production, is a CSG development in a laterally continuous coal seam that is separated from other aquifers by laterally continuous aquitards. The range of predictions provided in this product are valid as long as this general conceptual understanding holds. The largest discrepancy would arise if new information becomes available that indicates that aquitards separating the coal seams from aquifers containing model nodes are discontinuous in the vicinity of the CSG development, or, if new hydraulic property data become available that would warrant re-evaluating the role of different stratigraphic units as aquitards. The high degree of gas saturation of coal seams in the central Richmond river basin (Doig and Stanmore, 2012) suggests that an effective aquitard is in place that prevents leakage of gas or water. Additional information indicating that the coal seams are not laterally continuous would reduce the predicted impacts as the cone of depression will not spread as far laterally with less water needed to be extracted to achieve the same level of depressurisation in the coal seams.
2.6.2.8.2.2 Faults
Fault displacements of hydrostratigraphic units are neither represented in the three-dimensional geological model nor in the groundwater model. However, it is a well-known fact that faults are present in the Clarence-Moreton bioregion where significant vertical displacements of hydrostratigraphic layers occur (e.g. Ingram and Robinson, 1996; Raiber et al., 2016b).
A prevalent conceptualisation of the hydrodynamic behaviour of faults is that the disturbed zone parallel to the fault provides a conduit to flow vertically, while the shale gouge on the fault plane impedes flow horizontally. This can potentially compromise the integrity of aquitards. Without data from nested groundwater monitoring bores, there is no direct evidence to determine the significance of vertical groundwater fluxes between different hydrostratigraphic units within the Richmond river basin (Raiber et al., 2016b). Note that the high groundwater salinities in the alluvium near Coraki and the similarity between the course of Myrtle Creek and the orientation of Coraki Fault are an indication that this fault does act as a conduit for upward discharge of deep groundwater to shallow aquifers, or to the surface (companion product 2.3 for the Clarence‑Moreton bioregion (Raiber et al., 2016b)). However, this fault is located a considerable distance from the additional coal resource development, and it was also highlighted in companion product 2.3 that the Lamington Volcanics have a very dominant hydrological role in the Richmond river basin, which is likely to overwhelm the influence of faults in most areas. This indicates that at the regional scale, the influence of faults on predictions is likely to be relatively minor in most areas, yet it can be locally significant.
The lack of reliable data relevant to fault density and location is the main driver in the uncertainty related to faults and receives a ‘high’ score. Including faults in the three-dimensional geological model and the groundwater model was not feasible within this BA due to resourcing limitations and the timing as to when crucial relevant data for fault modelling (i.e. seismic data) was made available for use in the BA, hence the ‘medium’ score. Finally, explicitly representing faults in MODFLOW models, at least in MODFLOW 2005, as linear features that act as vertical conduits and horizontal barriers is not trivial and is technically challenging, hence the ‘medium’ score.
To reflect this uncertainty, the role of faults and their effect on predictions was assigned a ‘medium’ score, mostly because the effect is likely not to be regionally considerable, yet can be significant locally. Similar to effects of bore integrity (Doble et al., In press), the potential for regional drawdown in shallower aquifers induced by faults depends mainly on the density of faults and locally on the contrast between hydraulic conductivities of the fault zone and the aquifers. On a regional scale, Doble et al. (In press) show that the drawdown above an aquitard will only be noticeably affected by faults or failed bores when the density of faults is close to the ratio of the hydraulic conductivity of the aquitard and the fault zone. As an extreme example, assume that the vertical hydraulic conductivity of the fault zone is four orders of magnitude larger than the vertical hydraulic conductivity of the aquitard. In an area of 1 km2 this would require 10 faults with a length of 1 km and a width of 1 m before drawdown is noticeably affected. Although multiple regional fault systems and minor faults are known in the Clarence-Moreton Basin, the geological understanding of the Clarence-Moreton Basin indicates that such a fault density is unlikely. On a local scale, however, the flow through a fault is not only controlled by the hydraulic gradient across the fault and the hydraulic properties of the fault, but also by the hydraulic properties of the aquifers that the fault connects. Especially when the hydraulic conductivity of the lower aquifer is low, the flow rate through the fault will be controlled by the rate at which water can flow towards the fault, not by the rate that water can be transmitted through the fault. In the Clarence-Moreton bioregion, the main CSG target, the Walloon Coal Measures, has a low hydraulic conductivity especially at depth. It is therefore likely that the rate of water transmitted through a hitherto not known fault within the drawdown area of CSG is limited by the low hydraulic conductivity of the Walloon Coal Measures.
To formally assess the effect of faults on groundwater dynamics, faults will be incorporated in a future model realisation that will be developed as part of a CSIRO strategic funding project ‘Next generation methods and capability for multi-scale cumulative impact assessment and management’ (sub-theme ‘Tracer-based improvement of groundwater model conceptualisation and predictability’). This will allow a direct comparison between predictions derived from a faulted and an unfaulted model. Although this will further reduce uncertainty, it is important to note that the lack of deep and nested groundwater observation bores still leads to conceptual uncertainties on the hydraulic role of faults. This highlights the importance of installing nested groundwater monitoring bores in those areas.
2.6.2.8.2.3 Boundary conditions
The conceptual model necessitates the definition of several lateral and internal boundary conditions to groundwater flow, such as lateral general-head boundaries, the drainage of water from the top of the model to represent groundwater evapotranspiration and the major rivers.
The boundary conditions are assigned a ‘medium’ score for all three attributes, indicating that no single attribute dominates the choice and implementation of the boundary conditions. Additional data and resources will allow more detailed and complex representations of these boundary conditions. The resulting increased dimensionality, however, will increase the technical challenge of carrying out a comprehensive uncertainty analysis.
The overall effect of boundary conditions is scored ‘medium’. This score, however, needs to be nuanced as shown by the sensitivity analysis. The general-head boundary condition and recharge boundary condition have little effect on the change in groundwater level predictions. The riverbed conductance and drainage conductance of the top of model drainage boundary, can have an effect on predictions. A reduction in the uncertainty in these boundary conditions has the potential to at least partly reduce the uncertainty in the predictions.
2.6.2.8.2.4 Groundwater observation data
Groundwater level observations are often the only data used to constrain the parameters and conceptualisation of a groundwater model. In the groundwater model for the Clarence-Moreton bioregion groundwater level observations are used to constrain predictions in the top layer of the model.
In Section 2.6.2.7.1 the available groundwater observation data from the NSW Office of Water database in the groundwater model domain are presented and discussed. A large number of these observations date back to the late 1970s or early 1980s and mostly correspond to single water level readings carried out directly after completing a groundwater bore. The metadata associated with these measurements often indicate that the coordinates of the observation location are not surveyed, but estimated from a map. The elevation of ground level or the reference points for depth-to-watertable measurements is in the majority of cases not surveyed either, but estimated from maps or digital elevation models.
All of these observations are invaluable in building a conceptual understanding of groundwater flow in the region and identifying general trends in piezometric surface. Unfortunately, the uncertainties in spatial location and representativeness of measurements in groundwater bores shortly after installation greatly reduce the value of an observation in a formal comparison with the results of a groundwater model. Any observations that have no surveyed coordinates or are not carried out in groundwater observation wells are therefore excluded from the dataset used to constrain the groundwater model.
The amount of data available to constrain the groundwater model obviously scores ‘high’ in the data column (Table 9). A more extensive observation network will undoubtedly provide a stronger knowledge base to create and constrain groundwater models in this region. This issue receives a ‘medium’ score on the resources attribute. The quality control and assurance of the database entries, and their suitability to be included in the observation dataset to constrain the model, is entirely based on a desktop study based on the information provided in the database. A more comprehensive analysis of the original records or a field campaign to identify and verify spatial coordinates of the database entries has the potential to greatly reduce the uncertainty in the observation record. There are no technical issues for collecting, verifying or using groundwater level observations, hence the ‘low’ score for the technical attribute.
The final score on the effect on the predictions, despite the limited data availability and uncertainties in the observation record, is ‘medium’. This means this issue is important but not deemed to dominate the predictions. A larger observation database with less observation uncertainty has the potential to locally change the conceptual understanding of the system and change the final posterior parameter probability distributions. The sensitivity in Section 2.6.2.7.3, however, indicated that the changes in groundwater level predictions and surface water – groundwater flux are dominated by different parameters than the parameters to which the current groundwater level observations are sensitive. Constraining the parameters with additional groundwater level observations in the alluvium will therefore have limited effect on the predictions. The predictions of change in surface water – groundwater interactions are the main prediction. These predictions are sensitive to the riverbed conductance, which is a parameter that groundwater level observations close to river boundaries are sensitive to as well. It is very difficult, however, to constrain riverbed conductance greatly with groundwater level predictions as the groundwater levels will have a large influence from the recharge and river stage boundaries as well.
Predictions in the deeper layers are most sensitive to vertical hydraulic conductivity and storage. Any observations that have the potential to constrain these parameters, such as groundwater level observations in multi-level wells, time series of groundwater level in deeper layers or environmental tracers, will reduce the predictive uncertainty. Single or isolated groundwater level readings in the deeper layers will suffer from the same drawbacks as observations in the alluvial system. The observations have a lot of information on flow directions, but have little information on vertical gradients or storage properties.
2.6.2.8.2.5 Distance-based weighting of observations
Closely related to the previous assumption is the weights assigned to each groundwater level observation. The weight of an observation in constraining parameters for a particular prediction is based on the distance between observation and prediction and the distance of the observation to the nearest blue line network.
With the available data density and operational constraints, the development of a tailored weighting for each observation based on the aquifer it is situated in and the local hydrogeological conditions, is not possible. Therefore, the data and resources columns are scored ‘medium’. Technically it is trivial to implement a different weighting scheme, so the technical column scores ‘low’.
The overall effect on predictions is small, as the information in the groundwater level observations is not able to constrain the parameters relevant to the groundwater change predictions. Locally, however, the effect on predictions can be important such as in regions where none of the simulated groundwater levels are in agreement with the relevant observations and the model is not deemed reliable. The extent and shape of these regions is fully governed by the observation weighting function.
2.6.2.8.2.6 Zonal recharge from chloride mass balance
Groundwater recharge is implemented spatially in function of the outcropping geology as a correction factor to the temporal recharge signal obtained from the surface water model output. The correction factors are based on measurements of chloride in groundwater and rainfall with the chloride mass balance method (companion product 2.1-2.2 for the Clarence-Moreton bioregion (Raiber et al., 2016a)). The spatial coverage of bedrock groundwater chloride measurements in the Clarence-Moreton bioregion is variable, and there are only relatively limited data points in the Richmond river basin. Other reliable and representative measurements of diffuse recharge are not available in this bioregion.
More evenly distributed chloride measurements in groundwater observations across the outcropping geological units or other estimates of diffuse recharge will undoubtedly improve the zonation and parameterisation of groundwater recharge. For this reason, a ‘high’ score is attributed to the data column. It is unlikely that additional resources or different techniques will improve the recharge estimates based on the currently available data. Both these columns are therefore given a ‘low’ score.
Recharge estimates with reduced uncertainty will reduce uncertainty in groundwater level predictions; however, as the change in groundwater level is not sensitive to recharge, it will not, or only minimally, affect changes in groundwater level predictions. The effect on the predictions attribute is therefore scored ‘low’.
2.6.2.8.2.7 Spatial variability of hydraulic properties
The hydraulic properties of each hydrostratigraphic unit are implemented as spatially uniform horizontally although the hydraulic conductivity can vary with depth.
In comparison to the linked Surat Basin, the Clarence-Moreton Basin is a data-poor region, with very limited information on the hydraulic properties of key aquifers such as the Walloon Coal Measures and the overlying aquitards such as the Maclean Sandstone, Bungawalbin Member and Rapville Member. As outlined in Section 2.6.2.8.2.3, insufficient data are available to inform spatial variability at a regional scale. The data availability attribute therefore receives a ‘high’ score.
The level of spatial detail that can be accommodated in a numerical model is governed by the horizontal and vertical discretisation which, especially in the vertical direction, will always require an upscaling. This upscaling remains a challenging technical task with a wide variety of techniques available to upscale point measurements into equivalent hydraulic properties for a numerical model (Renard and de Marsily, 1997). The technical column is scored ‘medium’.
These technical challenges can be partly overcome through stochastic simulation of the hydraulic properties. The time and computational resources required to develop and apply stochastic hydraulic property simulators tailored to the bioregion are not available within the operational constraints of the Bioregional Assessment Programme. The resources column is therefore scored ‘medium’ as well.
The effect on the final predictions of the uncertainty in hydraulic properties is deemed to be moderate and is therefore scored ‘medium’. Any change in the hydraulic properties will affect the predictions directly, especially the deeper-layer storage and vertical hydraulic conductivity parameters. The wide prior distributions defined for the parameters ensure, however, that this uncertainty is adequately captured in the predictive distributions of drawdown and change of flux.
The validity of not accounting for spatial heterogeneity explicitly in the groundwater model hinges on the assumption that the representative elementary volume approach is valid at this scale and for these predictions. At the regional scale for groundwater quantity predictions, this is widely accepted to be the case as exemplified in guiding principle 7.3 in the Australian groundwater modelling guidelines (Barnett et al., 2012).
While introducing spatial heterogeneity might have a local effect in the changing extent of change in groundwater level predictions in the immediate vicinity of the largest stress, at larger scales the effect is minimal (see companion submethodology M07 for groundwater modelling (Crosbie et al., 2016)).
2.6.2.8.2.8 Prior parameter distributions
The prior parameter distributions are established by the Assessment team based on the available information for the Clarence-Moreton bioregion and equivalent analogue basins in Australia and the world.
Additional data will allow adjustment of these prior distributions to agree more closely with the conditions in the Clarence-Moreton bioregion. This warrants the ‘high’ score for the data component. Specifying prior distributions are not constrained by resources and there are no technical issues as the uncertainty analysis methodology is not prescriptive in the type of prior distribution used in the analysis. Both of these attributes score ‘low’.
The effect of the choice of parameter distributions is potentially important as there is little information available to constrain the majority of the parameters. Some of the most influential parameters, especially those controlling the CSG water production rate, are constrained only by the available production rate estimates. The posterior parameter distributions for these parameters are very similar to the prior distributions. The effect on predictions is therefore chosen to be scored ‘medium’.
To mitigate this, the distribution is chosen to be conservative, spanning at least two orders of magnitude for most of the hydraulic properties, as to ensure the predictive uncertainty is overestimated rather than underestimated.
2.6.2.8.2.9 Coal seam gas water production rates
In the groundwater model, CSG dewatering is implemented as a drainage boundary condition in the deepest model layer. Water is sourced from the entire layer, not from individual coal seams.
While non-trivial and challenging (Moore et al. 2015), it is technically possible, and within the resources of the BA, to implement a more detailed conceptualisation of the CSG depressurisation. However, insufficient data are available, both on the physical system and on the dimensions of the planned development, to adequately parameterise the added complexity. This motivates the scoring of ‘high’ on the data column with ‘low’ for resources and technical attributes.
One of the limitations of the current modelling approach is that it is not able to simulate dual-phase flow. Using a single-phase model is, however, likely to overestimate drawdowns and water extraction volumes (Herckenrath et al., 2015), in line with the precautionary principle. The codes do allow for specifying pumping rates, but these are not known and, because of the dual-phase aspect, will be unlikely to result in a drawdown that is representative of the depressurisation required for CSG extraction (see submethodology M07 (as listed in Table 1) for groundwater modelling (Crosbie et al., 2016)).
The effect of changing the water production rates on the prediction is large, as is shown in the sensitivity analysis, where most of the change in groundwater level predictions is very sensitive to the parameters related to the implementation of the CSG water production. A different configuration or number of wells or a change in the target depressurisation level (currently at 35 m above the top of the Walloon Coal Measures) has the potential to greatly change the predicted impacts. This explains the ‘high’ scoring for effect on predictions.
Constraining all parameter combinations with the estimated water production rates – at the very least – ensures that the predicted impacts are, to a degree, consistent, with the more detailed reservoir simulation carried out by the proponent.
2.6.2.8.2.10 Water usage for irrigation, stock and domestic
Metered-use data of groundwater pumping for irrigation, stock and domestic use were not available for the Clarence-Moreton team when the assessment was conducted. The pumping rates assigned to the model are therefore based on licensed use rather than actual use.
This assumption is driven by data availability, and scores ‘high’ in that column. Resources and technical attributes are scored ‘low’ as they do not form a limitation in implementing pumping rates.
The effect of water pumping for uses other than coal seam depressurisation on the prediction is deemed to be small as the pumping stress is considered identical under baseline and future development conditions. As the pumping volume is a small part of the overall water balance of the aquifer system, it is very unlikely that continued pumping is going to deplete the aquifers and cause non-linearities in the predicted change in groundwater levels. The overall score for the impact on predictions therefore is ‘low’.
2.6.2.8.2.11 Length of simulation period
Across the Bioregional Assessment Programme, the simulation period is chosen to be from 2012 to 2102 as discussed in companion submethodology M06 (as listed in Table 1) for surface water modelling (Viney, 2016) and companion submethodology M07 (as listed in Table 1) for groundwater modelling (Crosbie et al., 2016). For some parameter combinations and some model nodes this means that the additional drawdown is not realised within the simulation period.
Extending the simulation period is not limited by data as it is about the future, hence the score ‘low’. The resources attribute is, however, scored ‘high’. To ensure that the additional drawdown is realised at all model nodes for all parameter combinations, would require extending the simulation period with hundreds to even thousands of years. This would impose a sizeable increase in the computational demand and therefore compromise the comprehensive probabilistic assessment of predictions. The technical attribute is scored ‘medium’. It is trivial to extend the simulation period in the MODFLOW model. The climate scaling factors used to specify future rainfall and therefore recharge are not available beyond 2100. It is therefore a technical issue in devising a justifiable future climate to assign to the modelling.
The effect on predictions, however, is scored ‘low’, in line with the theoretical assessment of the relationship between dmax and tmax presented in submethodology M07 (as listed in Table 1) for groundwater modelling (Crosbie et al., 2016). It can be shown that any additional drawdown realised after 2102, will always be smaller than the additional drawdowns realised before 2102. This is in line with the precautionary principle as it means that by limiting the simulation period, the hydrological change will not be underestimated.
2.6.2.8.2.12 Only represent major rivers in groundwater model
Only major, mostly perennial, rivers are represented explicitly in the groundwater model as there is little doubt that these systems interact with the groundwater system. The connection status and level of interaction with the regional groundwater system of ephemeral, smaller streams are less well established. These are not explicitly represented and potential changes in flow rate are not assessed.
The data attribute scores ‘high’ as there is very little data available on historical flow rates in the higher order streams. The resources attribute also scores ‘high’ as incorporating this additional level of detail in a regional scale model is very time consuming. The technical component is scored ‘medium’ as a lot of the dynamics of these smaller features are greatly influenced by very local conditions, beyond the resolution of the grid. Accurately representing these features requires non-trivial upscaling of boundary conditions as pointed out by Brunner et al. (2010).
The overall impact on predictions is scored ‘low’. While the reduction in flow in smaller, ephemeral streams cannot be simulated. However, by not representing these surface water features, drawdown is over-estimated as they cannot be off-set by influx from surface water. Where the probability of drawdown is high at the location of an ephemeral stream, a more detailed study is warranted into the local surface water – groundwater dynamics.
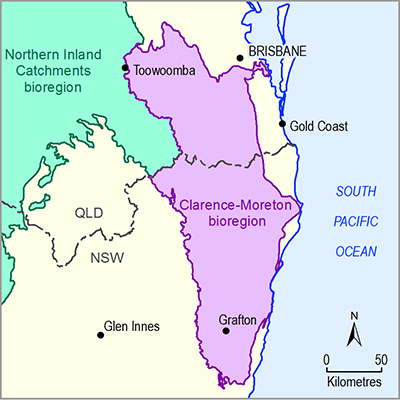
Product Finalisation date
- 2.6.2.1 Methods
- 2.6.2.2 Review of existing models
- 2.6.2.3 Model development
- 2.6.2.4 Boundary and initial conditions
- 2.6.2.5 Implementation of the coal resource development pathway
- 2.6.2.6 Parameterisation
- 2.6.2.7 Observations and predictions
- 2.6.2.8 Uncertainty analysis
- 2.6.2.9 Limitations and conclusions
- Citation
- Acknowledgements
- Contributors to the Technical Programme
- About this technical product