1.1.4.1.1 Hydrogeological characteristics of geological formations in the Hunter subregion
Hydrogeological investigations in the Hunter subregion have been undertaken for decades with earlier publications available to the project dating back to 1958 (Williamson, 1958), with some references from the early 1890s. These studies indicate that the hydrogeological systems in the subregion are largely influenced by bedrock origin and tectonic activities during the post-Carboniferous period (David et al., 2004; De Silva, 1998; WRC, 1986). These systems can be broadly grouped in three hydrogeological units: alluvial aquifers along major rivers and creek lines, coastal aquifers in the coastal area, and Triassic-Permian fractured rock aquifers of the Hunter subregion. These aquifer types are spatially variable and mostly localised.
Alluvial aquifers
The extent of alluvial deposits (Quaternary deposits) in the subregion is shown in Figure 37. They are commonly formed as sequences of clays, silts, sands and gravels (NSW Department of Planning, 2005), but are rarely uniform and in some areas have low permeability. Highly permeable coarse alluvial materials are frequently found in the base of the alluvial deposits (basal deposits). The deposits become finer towards the upper layers. Higher clay content in alluvial deposits, and hence lower permeability, is found in alluvium along the Goulburn River and its tributaries. This originates in the Liverpool Plains, and is said to be a by-product of the basalt weathering (McMahon, 1964; Williamson, 1958) (Figure 37). Further downstream where the rivers drain into clastic formations, the alluvial material is coarser.
The Hunter Valley Alluvium aquifer forms a groundwater management unit (GMU) with major importance for agriculture (DPI, 2005; 2013). The Kingdon Ponds and Dart Brook alluvial aquifers, located in the north-west of the subregion (Figure 37), are the major components of this GMU. Other important groundwater resources are found in the Page River, Wybong and Hunter River alluvial aquifers. The Page River alluvial aquifer is also associated with the most significant environmental values. Alluvial deposits developed along other creeks in the subregion are commonly thin and are not considered such important aquifers.
Hydraulic conductivity of the alluvial deposits is in the range of 10 m/day to 239 m/day (Williamson, 1958). Aquifer thickness in saturated zones of alluvium ranges from 3 to 17 m (Australian Groundwater Consultants Pty Ltd, 1984), and increases downstream. The watertable is shallow and within the first metres of the area is close to the river. The water levels are responsive to high rainfall and flooding events. During the drought between 2001 and 2004 the water levels in the Kingdon Ponds alluvial aquifer dropped by 5 m, which also led to an increase in salinity by 43% (NSW Department of Planning, 2005).
Data: Stewart and Adler (1995), Geoscience Australia (Dataset 1), Bureau of Meteorology, Climate and Water Division (Dataset 2)
Coastal sand aquifer
The Tomago Tomaree Stockton Coastal Sands groundwater is used for urban water supply by the Hunter Water Corporation (NSW Government, 2010), providing the potable water supply to the Newcastle area, and is particularly important during droughts (Woolley et al., 1995). However, during normal times, surface water catchments dominate potable water supplies to the Newcastle area. The Tomago sands extend 35 km from the Hunter River in the east to Port Stephens in the east and cover an area of 183 km2. Dunal and/or coastal (of aeolian origin) sands of medium grain size form a highly permeable unconfined aquifer and rainfall rapidly infiltrates through the unsaturated zone to recharge the saturated zone. This formation receives the highest diffuse recharge rate in the region. However the rapid infiltration makes this aquifer susceptible to contamination and so to ensure the protection of the quality of the water resource the majority of the aquifer is protected as a water reserve (Crosbie, 2003).
Transmissivity was estimated between 400 m/day to more than 600 m/day (Crosbie, 2003) with a specific yield of about 0.2. The groundwater level is very responsive to rainfall events, with groundwater level rises over a metre observed on an event basis (Crosbie, 2003).
A significant proportion of the area at Tomago has been listed as a high priority groundwater-dependent ecosystem (DIPNR, 2003).
This aquifer contains mineral sands and has been investigated for its mineral deposits as well as being a source of water (Coffey and Sinclair Knight Mertz, 1996; SML, 1971).
Fractured rock aquifers
There is a series of aquifers hosted by fractured rock formations, including the Liverpool Range Volcanics, Jurassic, Narrabeen and Permian group. The Sydney Basin is dominated by porous rocks that are fractured, and pumping yield from these rocks is generally low.
Liverpool Range Volcanics
The north-west area of the Hunter subregion is formed by Cenozoic formation associated with alkaline mafic to ultramafic flows (basalt, dolerite), polymictic conglomerate and quartzose sandstone. This formation thickness varies from 800 m (Barnes et al., 2002) to less than 100 m in Wybong Creek catchment (Kellett et al., 1989).
The basalt terrain can be quite productive in terms of groundwater (De Silva, 1998). The highest yields occur where a combination of cavities are interconnected with joints, fractures and faults (NSW Department of Planning, 2005). Other aquifers associated with the basalt can form due to weathering and erosion on the surface of the lava sheet prior to subsequent lava flows. The water quality associated with basaltic terrain is generally good with low salinity. However, in some locations the water may exhibit hardness due to calcium and magnesium carbonates or may be slightly acidic and contain iron. Bore yields in the Cenozoic aquifer are typically 432 m3/day (NSW Department of Planning, 2005).
Terrestrial deposits of Jurassic and Triassic period (Narrabeen Group)
In some publications (De Silva, 1998), the water-bearing strata in terrestrial units of the Carboniferous period and Triassic period (Narrabeen group) are characterised as porous aquifers, implying that hydraulic properties in units of these groups are associated with primary porosity. These water-bearing units can be separated into an individual group as groundwater here has certain specific properties compared to other bedrock aquifers (referred to as fractured rock).
The Jurassic formation, mainly represented by Pilliga Sandstone, is only present in the north-west of the subregion and consists of medium to very coarse grained sandstone and conglomerate. Only limited information on hydrogeological characteristics is available, mainly associated with data generated for the Ulan Mine Complex. Here Jurassic Pilliga Sandstone, overlays the Narrabeen Group. Both units, along with the underlining Permian coal measures, form an aquifer. Compared with other units, the hydraulic conductivity of the Jurassic rock is lower but also more variable. Within the mining area the Jurassic sandstones and siltstones are also mostly unsaturated.
The Narrabeen Group covers a large area in the south and south-west of the Hunter subregion. This formation is comprised of conglomerates, sandstones and shales deposited in fluviatile, fluviatile-deltaic and lacustrine environments with overall thickness ranging from 90 to 700 m (Moffitt, 2000). The Narrabeen Group forms a locally significant aquifer and springs, particularly in the Blue Mountains area.
The Narrabeen Group forms a sub-horizontal sedimentary sequence of aquifers and aquitards. Generally the sandstone units act as aquifers and the claystone units act as aquitards. Permeable zones of these units are occasionally associated with coarser grained materials containing relatively higher pore space. However, secondary porosity features, such as bedding plane partings and the network of joints, are the predominant flow pathways. It is generally accepted that hydraulic conductivity is an order of magnitude greater in the horizontal direction (Ward and Kelly, 2013, p. 42, Table 5.1). However, some vertical fluxes through the fracture network are possible locally (McNally and Evans, 2007). Bores yields are generally in the range of 17 to 216 m3/day (NSW Department of Planning, 2005). On average hydraulic conductivity of sandstone aquifers is 0.01 m/day with effective porosity of 0.02 (Kellett et al., 1989). Changes in hydraulic conductivity of these units with depth are characterised by a logarithmic decrease in conductivity with linear decrease in depth, reaching less than 10-4 m/day at 400 m below ground (see Ward and Kelly, 2013, p. 22, Figure 3.5).
Groundwater discharge from the sandstone units of the Narrabeen Group contributes to baseflow in the streams and to supporting groundwater-dependent ecosystems.
Permian Group
The Permian units are largely associated with a series of coal measures. They are commonly subdivided into the upper and lower coal measures and the intervening marine sequence. The saline water associated with this geological unit is thought to have a controlling influence on the overall water quality of the Hunter River (Kellett et al., 1989). The fine-grained, consolidated nature of these rocks is reflected in low primary porosity, with most groundwater flow associated with secondary faults, fractures and joints. The coal seams exhibit many joints and cleats and are the main aquifers within these rocks.
Parts of the overlying weathered zone or regolith act as an intergranular aquifer storage. These zones may feed into springs following periods of high rainfall but most are depleted during extended dry and drought periods. Watertables in the regolith can be isolated from deeper coal measures through the presence of massive and relatively impermeable conglomerates that overlie the coal seams. This isolation is however likely to be interrupted at locations where vertical faulting provides a connecting pathway to deeper strata (Mackie Environmental Research, 2006).
The most transmissive fractured rock aquifers are likely to be those with the most and youngest open tension fractures, where the likelihood of lining and filling with mineral precipitates is lower. Such areas were identified by Kellett et al. (1989) (in addition to the face of the escarpment of Triassic rocks):
- the Permian rocks of the Hunter River valley floor as intersecting sets of closely spaced cleats perpendicular to bedding in coal seams
- fractures associated with the youngest, low angle joints and bedding plane separation in the Permian rocks of the valley
- the hinge areas and limbs of anticlines (e.g. Muswellbrook anticline) where two sets of primary tension fractures (parallel and perpendicular to the fold axes) were formed; the further lateral extension of fractured zone were associated with later uplift of domal structures (see map in Section 1.1.3.3).
The hydraulic properties in these areas could be an order of magnitude greater than in other regolith within the subregion.
A reduction in the hydraulic conductivity of the coal seam aquifers with depth is observed in many coal mines. Australian Groundwater Consultants Pty Ltd (1984) developed an equation based on the interpretation of depth-dependent hydraulic conductivities of 17 coal seams in the Upper Hunter Valley as:
|
(1) |
where k is the hydraulic conductivity (m/day), k0 is the reference hydraulic conductivity of 5 m/day, c is the slope of the trend line (0.046 for Hunter Valley coal seams) and z is depth below ground (m).
Aquifers in the porous rocks within the Permian sandstones, shales and coal measures strata are hydraulically connected with the alluvial aquifers. It is acknowledged that hydraulic connectivity between the near-surface regolith aquifers and groundwater in the coal measures is not well characterised (Ward and Kelly, 2013). However groundwater exchange in the deeper strata is not significant. The groundwater ages of 22,000 to 33,000 years reported by McLean et al. (2010) for the Blakefield seam 323 m below ground level are consistent with the millennial rates of fluid movement expected in porous rock at these depths.
Depth to groundwater is greater in the elevated part of the landscape and becomes shallower in proximity to streamlines. As such, the groundwater flow directions in shallow hydrogeological systems, regolith and alluvial aquifers, largely follow similar pathways as surface water following topographic features in the subregion.
1.1.4.1.2 Structural elements and their hydrogeological characteristics
In addition to the effect of folding on the hydraulic properties of Permian units, there is evidence that faults play an important role in the hydraulic connectivity of fractured rock and alluvial aquifers at some locations. Increased alluvial aquifer salinity was associated with the Mount Ogilvie Fault area (Kellett et al., 1989). At the same time, the Hunter Thrust Fault is considered to be an impermeable boundary between the New England Fold Belt, in the north-east, and the Sydney Basin.
1.1.4.1.3 Groundwater use
The NSW Office of Water is responsible for the development and review of water sharing plans (WSP) which govern the extraction of water from surface and groundwater sources in NSW. All WSPs are a regulation of the NSW Water Management Act 2000 and have a term of ten years from the date of commencement, after which time they must be extended or replaced[1].
Alluvial and non alluvial aquifers are present in the Hunter subregion and groundwater extraction from these aquifers is used for a range of purposes including domestic, stock, irrigation, town water supply and industrial purposes, with a proportion of water protected for the environment in all water sources.
Groundwater extraction limits in WSPs are based on the entitlement when the plan commenced. Where a WSP is in place, entitlement for general purposes can only be purchased on the market. Identified specific purposes can apply if provided for by the relevant WSP or under the NSW Water Management (General) Regulation 2011. In areas where a WSP has not commenced, access licences may still be applied for under the NSW Water Act 1912.
Alluvial aquifers identified in the Hunter subregion are considered to be highly connected to surface water, and are generally managed conjunctively with the surface water source they are associated with. Most of the alluvial groundwater in the Hunter subregion is managed under the NSW Water Sharing Plan for the Hunter Unregulated and Alluvial Water Sources 2009 for the Hunter Unregulated and Alluvial Water Sources. This WSP may be amended in the future to include floodplain alluvium which does not have a strong connection to surface waters.
The draft WSP for the North Coast Fractured and Porous Rock Groundwater Sources, which is currently under development with a view to commence in 2015, will manage groundwater extracted from the hard rock groundwater sources which fall within the Hunter subregion.
Table 10 gives an estimate of groundwater entitlement in these two WSP areas.
Table 10 Groundwater entitlements
The boundaries of the areas covered by these water sharing plans extend beyond the Hunter subregion.
adenotes that only a portion of the water source falls within the Hunter subregion.
bdenotes that this water source is set to be merged in 2015 with the water sharing plan for the Hunter Unregulated and Alluvial Water Sources.
The key water sources for the NSW Water Sharing Plan for the Hunter Unregulated and Alluvial Water Sources 2009 are the Hunter Regulated River Alluvial Water Source, the Dart Brook Water Source, the Pages River Water Source, the Bylong River Water Source and the Lower Wollombi Brook Water Source. The Kingdon Pond Alluvium is part of the Dart Brook Water Source.
While there is minimal coastal sand aquifers that fall within the Hunter subregion, a large portion of the Tomago and Stockton groundwater sources do fall within the Hunter subregion. Estimated groundwater share components in these groundwater sources are 26,090 and 1008 respectively, with most of the entitlement in the Tomago water source being for the purpose of town water supply.
Generally, one share component is equivalent to 1 ML/year unless an available water determination (AWD; the volume of water available to licence holders for any particular water year) of anything other than 1 ML/share is issued. AWDs of less than 1 ML/share may be issued for aquifer access licences under WSPs to ensure compliance with the long term average annual extraction limits (LTAAEL) identified by the relevant WSP.
There are small portions of other WSP areas that fall within the Hunter subregion – however groundwater entitlement in these areas is relatively minor.
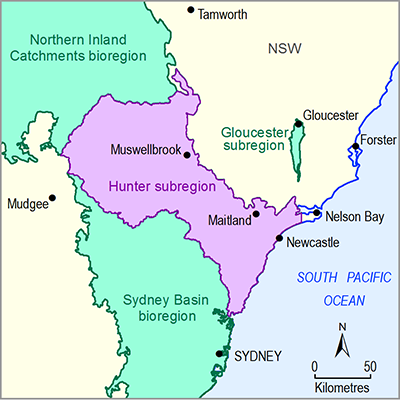