- Home
- Assessments
- Bioregional Assessment Program
- Clarence-Moreton bioregion
- 2.3 Conceptual modelling for the Clarence-Moreton bioregion
- 2.3.2 Summary of key system components, processes and interactions
- 2.3.2.6 Conceptual uncertainties of geology and hydrogeology
2.3.2.6.1 Three-dimensional geological model uncertainty
Three-dimensional geological models are created from datasets, such as drill-hole and geophysical data, which sample the subsurface at a limited number of locations, and with variable quality resolution. These data constraints mean that all geological models contain some degree of inherent uncertainty (e.g. Wellmann et al., 2010; Raiber et al., 2012). Furthermore, there is commonly a high degree of randomness as to where these subsurface data are gathered from, as many petroleum, mineral or groundwater resource exploration programs focus on small areas where a dense network of bores or wells is drilled. Consequently, and based on the available data, different three-dimensional geological model realisations can be developed from the same input data. In general, uncertainties in three-dimensional geological models are typically related to (e.g. Mann, 1993; Davis, 2002; Raiber et al., 2012):
- data density
- data quality
- geological complexity
- geological interpretations and conceptual uncertainties.
Some simplifications are always needed in three-dimensional geological models. In the Clarence-Moreton bioregion, such simplifications mean, for example, that stratigraphic units that only occur locally or have poorly understood relationships to other units (e.g. Woodenbong Beds), are not identified as separate model units. In addition, no differentiation was made in the Clarence-Moreton three-dimensional geological model between different types of Quaternary sediments (e.g. alluvial sediments, coastal sands or shallow marine sediments) in the coastal regions of the Richmond and Clarence river basins. These differences cannot be resolved at the regional scale of the models developed for this BA, and would require small-scale models that specifically focus on these areas.
Selected stratigraphic sections (SS) are shown to give examples on variations of geological uncertainty across the Clarence-Moreton bioregion. Selected major faults and other structural elements are projected as vertical surfaces on the three-dimensional geological model.
Data: Bioregional Assessment Programme (Dataset 1, Dataset 2, Dataset 3, Dataset 4); Australian Geological Survey Organisation (Dataset 13)
In this section, the spatial distribution of uncertainties of the three-dimensional geological model of the Clarence-Moreton bioregion, which was related to data density, data quality, geological complexity and geological uncertainties, is explained using multiple stratigraphic columns (Figure 29). These stratigraphic columns show the distribution of the stratigraphic units in various parts of the Clarence-Moreton bioregion together with a qualitative assessment of the confidence in the accuracy of layer boundaries varying from high, medium or low. This qualitative assessment is based on the availability of different data sources (e.g. groundwater bores, exploration wells or seismic data; Raiber et al., 2016) and how they characterise the geometry of different geological layer boundaries (which define the contact between geological formations). In high confidence areas, the three-dimensional geological model is likely to predict the depth where different stratigraphic units are intersected (i.e. formation tops) with a relatively high degree of accuracy. Conversely, where the confidence level is low, the discrepancy between the predicted and actual depth of formation top intersections observed at a new well that would be drilled at the same location could potentially be very high. Stratigraphic columns are described below:
- Stratigraphic column SC1: at this location in the Lockyer Valley, the density of groundwater bores in the alluvium and deeper aquifers is very high. As a result, the accuracy of the layer boundaries between the alluvium and the underlying Gatton Sandstone, and between the Gatton Sandstone and the underlying Woogaroo Subgroup, is classified as high. As there are fewer groundwater bores or exploration wells that intersect the interface between the Woogaroo Subgroup and the underlying basement, the confidence level in this boundary is classified as medium. Overall, the aquifer geometry in this area is well understood, and additional data from future groundwater bores or exploration wells are unlikely to result in significant changes to the three-dimensional geological model.
- Stratigraphic column SC2: in this area within the headwaters of the Bremer river basin and at the crest of the Great Dividing Range, there are some groundwater bores that intersect the interface between the Main Range Volcanics and the Walloon Coal Measures, but there are no groundwater bores or exploration or stratigraphic wells that intersect any of the deeper units. Consequently, the confidence level in layer boundaries is classified as low, and multiple three-dimensional geological model realisations are possible which all honour the available data. The different model realisations could also translate into very different conceptual hydrogeological models (e.g. with regards to the location of groundwater divide). However, as this area is remote from the Richmond river basin and the West Casino Gas Project area and is not hydraulic connected (Figure 13), this does not have any influence on the predictions of the Richmond river basin groundwater model.
- Stratigraphic column SC3: this area in the Richmond river basin, which forms part of the West Casino Gas Project area, is part of the Casino Trough that has considerable structural complexity. The East Richmond Fault, one of the major faults where stratigraphic units are displaced by several hundred metres (e.g. Ingram et al., 1996), is located to the west of this area. The fault-bound mid-basin high is located to the east of this area. However, there are many CSG and petroleum exploration wells with high quality stratigraphic data, as well as many seismic reflection lines (companion product 2.1-2.2 for the Clarence-Moreton bioregion (Raiber et al., 2016)) in this area. Consequently, and despite the structural complexity, the confidence in the current aquifer geometry and the reliability of the identified layer interfaces in the area of the West Casino Gas Project are both high. A three-dimensional geological model realisation that represents fault displacement of stratigraphic units will further help to more accurately represent aquifer geometry. However, additional stratigraphic data from new wells would unlikely result in any fundamental changes to the current conceptual model.
- Stratigraphic column SC4: there is considerable structural complexity in this area with a relatively good coverage of seismic lines (companion product 2.1-2.2 of the Clarence-Moreton bioregion (Raiber et al., 2016)). However, there are very few exploration wells, and hence only limited well control of the seismic data, which may lead to uncertainties in seismic travel time to depth conversions. As outlined in companion product 2.1-2.2 of the Clarence-Moreton bioregion (Raiber et al., 2016), there are very few groundwater bores in this area, and hence there is low confidence in the modelled depth of the interface between the alluvium and the sedimentary bedrock, as well as the interface depths between different sedimentary bedrock units. Additional data that may arise from future wells would considerably improve understanding of aquifer geometry and could result in fundamentally different model realisations. Due to the predominantly west-to-east direction of groundwater flow and the considerable distance from the West Casino Gas Project, this low confidence is unlikely to influence predictions of the Richmond river basin groundwater model.
- Stratigraphic column SC5: this area, which is part of the Warrill Creek Syncline in the Bremer river basin, has a very good coverage of CSG exploration wells (Figure 29 and companion product 2.1-2.2 (observations analysis, statistical analysis and interpolation) of the Clarence-Moreton Bioregional Assessment (Raiber et al., 2016)). Most of these wells intersect the Walloon Coal Measures and Koukandowie Formation interface, but are not deep enough to intersect the contact between the Koukandowie Formation and the Gatton Sandstone. Consequently, the interfaces between the Walloon Coal Measures and the Koukandowie Formation are well understood, but there is limited knowledge on deeper stratigraphic unit boundaries. Additional deep wells would substantially increase the level of confidence.
- Stratigraphic column SC6: this location close to the border between Queensland and NSW represents the area within the Clarence-Moreton bioregion where the three-dimensional geological model has the lowest level of confidence. As a result of the large thickness of the Lamington Volcanics and the high relief topographic surface at the boundary between the Richmond River and the Logan-Albert river basins, there are no stratigraphic wells, exploration wells or groundwater bores that are deep enough to intersect the different sedimentary bedrock units underneath the Lamington Volcanics. There are basement highs to the east (Mount Warning) and west (Mount Barny). Elsewhere within the Clarence-Moreton Basin, the thickest sequences of basalts occur in areas where basement highs are present. However, based on the available evidence, it is not possible to determine whether the Casino Trough in NSW and the depositional centre near Beaudesert are hydraulically linked, or if there is a basement high separating them (Figure 13 and Figure 29). Due to limited data in this area, the three-dimensional geological model contains significant qualitative and subjective interpretations, and fundamentally different model realisations are possible. Hence, a single new deep well in this area would substantially increase the confidence in identifying aquifer geometry and layer boundary locations. However, there is a much higher level of confidence in the reliability of the layer boundaries and particularly the intersection between the Lamington Volcanics and the underlying sedimentary bedrock to the south (towards Kyogle and the West Casino Gas Project area) and the south-east (towards Lismore and the Alstonville Plateau).
- Stratigraphic column SC7: there is a relatively good coverage of exploration wells and groundwater bores in this area (Figure 29 and companion product 2.1-2.2 for the Clarence-Moreton bioregion (Raiber et al., 2016)). However, considerable structural complexity exists, which includes juxtaposition (vertical displacement) of aquifers and aquitards of potentially several hundred metres along the Coraki Fault (Figure 29). Furthermore, this area is located close to the margin of the Clarence-Moreton Basin, where layers dip steeply. Consequently, despite the existence of multiple exploration wells and seismic data in this area, the confidence in the identified layer boundaries at this location has been classified as low. Additional data would increase confidence in the three-dimensional geological model predictions and shed further light on how faults may influence groundwater flow.
Although outside of the scope of this BA, the understanding of conceptual geological uncertainties can be refined further in a more quantitative way. For example, formation top intersections from selected wells can be omitted when building the three-dimensional geological model, and the goodness-of-fit between the predicted formation tops and the actual formation top known from the well can be compared (Raiber et al., 2012). Different approaches of characterising and quantifying uncertainty in three-dimensional geological models have been proposed, for example, by Wellmann and Regenauer-Lieb (2012) and Lindsay et al. (2012).
Unlike groundwater models which are commonly developed to simulate transient groundwater behaviour, most three-dimensional geological models are atemporal. However, such models should not be considered as a static final product. Instead, they should be part of a dynamic learning process in which the three-dimensional geological model is used as a guide to target future optimal sampling locations (i.e. locations most in need of new input data such as new wells or seismic data). In this way, any newly acquired data can be input back into the model, thereby leading to improved three-dimensional geological model realisations.
2.3.2.6.2 Conceptual hydrogeological model uncertainty – what is known and unknown
The conceptual hydrogeological model provides the basic understanding of how hydrogeological system components and processes operate and interact (Bredehoeft, 2005). In this product, multiple lines of evidence were used to develop a conceptual understanding of how key system components in geology, hydrogeology and surface water interact in different parts of the Clarence-Moreton bioregion (with a focus on the Richmond river basin). In this section, an overview is provided to identify parts of the conceptual hydrogeological model that are well understood, and highlight areas of major conceptual uncertainty.
Some of the geological uncertainties at selected locations within the Clarence-Moreton bioregion (Figure 29) impact the conceptual hydrogeological model and ultimately contribute to the uncertainty of the groundwater model, which is described in companion product 2.6.2 for the Clarence-Moreton bioregion (Cui et al., 2016b). As indicated, multiple three-dimensional geological model realisations are possible based on currently available data for the Clarence-Moreton bioregion. This conceptual geological uncertainty can lead to an ‘element of surprise’ in the hydrogeological conceptual model (Bredehoeft, 2005). This applies especially to the area near the crest of the Lamington Volcanics at the northern margin of the Richmond river basin of the groundwater model domain (stratigraphic column 6 in Figure 29). In this area, the uncertainty is very high due to a lack of groundwater bores and exploration wells, attributed to the steep topographic relief and the considerable thickness of the basalts (inferred basalt thickness of up to approximately 850 m). However, as shown in Figure 29, the confidence in the three-dimensional geological model within the Clarence-Moreton bioregion is highest in the area of the West Casino Gas Project due to extensive coverage of exploration wells and seismic data. This means that although there are many geological uncertainties across the entire Clarence-Moreton bioregion, including poor stratigraphic characterisation in some regions, the confidence level in aquifer geometry and the presence and continuity of aquitards is much greater in the West Casino Gas Project area. Consequently, the central part of the groundwater numerical modelling domain coincides with the highest level of three-dimensional geological model reliability in the Clarence-Moreton bioregion.
Where rocks of contrasting hydraulic properties (e.g. aquifers and aquitards) are juxtaposed, faults can form barriers and/or preferential pathways for groundwater flow, which can lead to compartmentalisation of the regional groundwater flow systems (Raiber et al., 2015). The locations and orientation of major faults in the Richmond river basin are well known (e.g. Ingram and Robinson, 1996; Rassam et al., 2014; Raiber et al., 2016), and unlike elsewhere in the Clarence-Moreton bioregion, it is unlikely that there are any major regional fault systems where significant fault displacements occur that have not yet been identified. Well log and seismic data suggest that there is some degree of juxtaposition of aquifers and aquitards along major faults in the Richmond river basin, especially at the Coraki Fault and at the East Richmond Fault (Figure 29). Furthermore, there is evidence from the orientation of streams and groundwater chemistry data that the Coraki Fault may act as a partial groundwater flow path to the surface. Within available time and resources, no faulted three-dimensional geological model could be developed as part of the Clarence-Moreton Bioregional Assessment (Raiber et al., 2016). This limitation will be addressed as part of a CSIRO strategic project (‘Next generation methods and capability for multi-scale cumulative impact assessment and management’ (sub-theme ‘Tracer-based improvement of groundwater model conceptualisation and predictability’)). Another part of this strategic project is to use remote-sensing data to confirm whether there is any surface expression of the major faults. Environmental tracer sampling will be conducted at locations where discharge of deep groundwater to the surface is inferred based on the currently available evidence. However, the lack of deep and nested groundwater observation bores means that although this additional work will help to considerably reduce uncertainty, it will not be possible to remove all conceptual uncertainties on the hydraulic role of faults. Ultimately, to further reduce this uncertainty, nested groundwater monitoring bore sites are required.
Apart from the geological uncertainty, a major source of uncertainty in the conceptual hydrogeological models that can directly translate to uncertainties in the groundwater model is the rate and spatial variability of groundwater recharge. An evaluation of recharge was carried out as part of this BA (Figure 12). This recharge assessment is associated with uncertainties related to the spatial heterogeneity of geological materials, climatic variability and the scarcity of climatic and hydrogeological data, as highlighted in companion product 2.1-2.2 for the Clarence-Moreton bioregion (Raiber et al., 2016) and in many scientific publications (e.g. Scanlon et al., 2002). However, despite these uncertainties, the role of the Lamington Volcanics as the major recharge area within the Richmond river basin, where recharge rates are at least an order of magnitude higher than recharge rates to the sedimentary bedrock, is well understood. Furthermore, it is supported by multiple lines of evidence discussed earlier in this section, such as groundwater chemistry data, stream flow rates and general understanding of the link between the alluvial aquifers and the Lamington Volcanics.
It is also well understood that the Lamington Volcanics are simultaneously a major discharge area (Brodie and Green, 2002), where a large proportion of the initial recharge discharges into the stream locally with short lag times and following short flow paths. Only a small proportion of this recharge percolates to deeper aquifers. Hence, there are many springs feeding streams and generating most of the stream flow in the Richmond river basin (Figure 27). This is also documented by multiple lines of evidence, such as water chemistry data, stream flow rates and the association of springs and other groundwater-dependent ecosystems (GDEs) with this feature. Furthermore, the critical hydrological significance of the volcanics as the source for much of the current surface water and groundwater extraction in the Richmond river basin is also underpinned by analogues from other catchments within the Clarence-Moreton bioregion (e.g. Lockyer Valley or Bremer river basin) where more data are available.
A further source of uncertainty in the conceptual hydrogeological model relates to the connection between aquifers and aquitards. Hydraulic connection between aquifers can be controlled to varying degrees by geological structures (discussed above), aquifer composition, aquifer geometry and internal aquifer architecture (e.g. Raiber et al., 2015). In the context of aquifer connectivity in the Richmond river basin, the main uncertainty is related to the lack of petrophysical data of aquitards. In the West Casino Gas Project area, stratigraphic data from the dense network of CSG exploration wells indicate that the aquitards are laterally continuous. If inferred aquitards such as the Maclean Sandstone and the Bungawalbin Member are an ideal regional seal, then they should limit or prevent the vertical hydraulic connection between the overlying and underlying stratigraphic units (i.e. between the Walloon Coal Measures and shallower aquifers) within the West Casino Gas Project area. The high gas saturation levels described by Doig and Stanmore (2012) confirm that there is an effective seal in place in the central part of the Richmond river basin near the West Casino Gas Project. However, even if there are effective aquitards present that limit propagation of impacts associated with the depressurisation of the Walloon Coal Measures to shallower aquifers or the surface within the West Casino Gas Project area, there can still be connectivity elsewhere. For example, where the sedimentary bedrock units thin and pinch out at the surface or where they subcrop underneath the alluvium at the down-gradient end of long regional flow paths at the eastern margin of the Clarence-Moreton bioregion in the Richmond river basin, there is likely to be upwards discharge of deep groundwater to shallow aquifers or to the surface. Without data from nested groundwater monitoring bores, there is no direct evidence to determine to what extent vertical groundwater fluxes between different hydrostratigraphic units occur within the Richmond river basin. However, auxiliary evidence from hydrochemical data (i.e. the freshness of most alluvial groundwaters at the eastern boundary of the Clarence-Moreton Basin) within the Richmond river basin and other catchments within the Clarence-Moreton bioregion (Section 2.3.2.2.4) suggests that discharge from sedimentary bedrock is not the major source of water for alluvial aquifers. In most areas, this sedimentary bedrock discharge component is likely to be overwhelmed by other sources such as the contribution from the Lamington Volcanics or surface water recharge during ‘normal’ (i.e. non-drought) climatic periods, but may become more important in some areas during droughts.
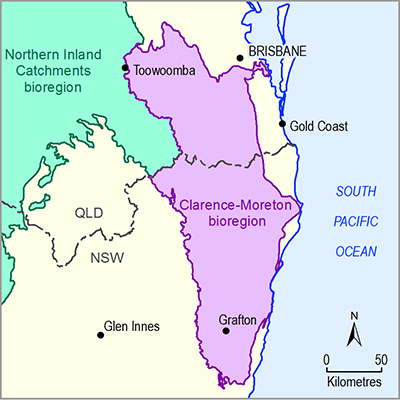