4.1 Data, information and model acquisition
4.1.1 Assessment of existing data extent and quality
Geological and hydrogeological data will primarily be obtained from holes drilled for conventional hydrocarbons, CSG exploration, mineral exploration, groundwater extraction, coal exploration and stratigraphic tests. Basins within the bioregions of interest have as few as 14 wells (Pedirka Basin) to as many as several thousand (Surat Basin). Recent database listings at Geoscience Australia show nearly 4000 conventional petroleum wells and stratigraphic holes and over 5000 CSG wells in the areas of interest, mostly in the Surat, Bowen, Clarence-Moreton and Gunnedah basins. It is expected that a significant number of new observations will be needed for each BA. Wells will have to be selected on the basis of spatial distribution and data quality. Wireline log data will need to be analysed to provide additional data on rock types, porosity, permeability and coal distribution.
In spite of the large number of drill holes, there are still major gaps in areas not considered prospective in the past. In some areas, seismic reflection data may be needed to map basin structure and unit thickness and, in others, outcrop data will be needed. Alternative geophysical techniques, such as time-domain airborne electromagnetics, may also be applicable. The method will depend on the geology and the salinity of groundwater.
4.1.2 Use of existing syntheses in bioregional assessments
Existing syntheses of data and information in the bioregion or sub-regions of the BA should be accessed, such as:
- Permian coals of Eastern Australia (Harrington et al., 1984)
- Geology and petroleum potential of the Clarence-Moreton Basin (Wells and O'Brien, 1994) and the Namoi Catchment Water Study (Schlumberger, 2012)
- Geology of the Surat Basin in Queensland (Exon, 1976)
- Great Artesian Basin Water Resource Assessment www.csiro.au/Organisation-Structure/Flagships/Water-for-a-Healthy-Country-Flagship/Sustainable-Yields-Projects/Great-Artesian-Basin-Assessment.aspx
- Healthy HeadWaters program in the Upper Condamine www.nrm.qld.gov.au/water/health/healthy-headwaters/index.html.
4.1.3 Cost–benefit considerations of data and modelling
Undertaking a BA will always entail consideration of the marginal benefit versus cost associated with the time and price of project components. A balance must be achieved between costs, complex model formulation and simulation, data acquisition and processing, and accuracy of model output and impact analyses. This balance requires exercising judgment that must be recorded in the workflow. During the contextual information component of the BA, consideration must be given to the costs of modelling and measurement relative to risks associated with water-dependent receptors and assets, which bears on the appropriate level of detail required in any bioregion. It is likely that that amount of knowledge is typically the limiting factor in any BA and investments in different components should maximise the marginal benefit relative to cost (i.e. maximally reduce uncertainties). Decisions made at each point must be recorded in the workflow and supported by reasons. The assembly of datasets as part of the data register and identification of data gaps must include, to the best estimate possible given the extent of knowledge within a bioregion, an assessment of how new data is to be used in the analysis and how important those data are in determining impacts of CSG and coal mining development on water-dependent receptors and assets. In the case of data gaps, a priority ranking on new datasets is required that takes into account, to the extent possible in the experience of the agency conducting the research, the cost and benefit of obtaining those data in relation to the goals of the BA. Where models need to be developed or adapted to undertake quantitative uncertainty analyses, an assessment of the degree of development of these analyses relative to best practice and the assigned time frame of the project needs to be made. An appropriate achievable end-point for this development must be identified within the project time frame and recorded in the workflow. The workflow must also identify where future revisions of the BA would modify, advance or improve current analyses.
4.1.4 Relevant existing models, maps, datasets and monitoring
4.1.4.1 Data
Commonwealth agencies collaborate with states and territories to maintain national geological, topographic and biological spatial data as part of the Australian Spatial Data Infrastructure. Examples of databases containing relevant information are:
- OZMIN: Geoscience Australia maintains the OZMIN database that contains coal reserves and coal resource estimates and spatial data relating to deposits. The database contains commercially sensitive information but outputs of non-sensitive information will be possible and useful.
- PEDIN: Geoscience Australia maintains a national database of petroleum wells containing basic metadata such as location, operator and total depths. This database links to other databases of stratigraphic information (Stratdat), organic geochemistry (ORGCHEM) and petrophysical analyses (RESFSCS). Wells for which metadata are not available in existing databases (PEDIN) will need to be loaded and then transferred to GIS for mapping and spatial analysis and the geophysical logs loaded into the analysis systems.
- AWRIS: The Australian Water Resources Information System (AWRIS) is a national database of water information that is supplied to the Bureau of Meteorology by over 200 organisations across Australia, as defined in the Regulations to the Commonwealth's Water Act 2007. The Bureau collects 75 variables across ten categories of water information, including streamflow, groundwater, climate, water storages, water entitlements, allocations, trades, restrictions and use.
- Geofabric: The Australian Hydrological Geospatial Fabric (Geofabric) is a framework for discovering, querying, reporting and modelling water information. It is a specialised geographic information system (GIS) that registers the spatial relationships between important hydrological features such as rivers, water bodies, aquifers, monitoring points and catchments. The Geofabric currently defines national surface water hydrology at 1:250,000 scale and groundwater aquifers at 1:1,000,000 scale. Future releases will improve the surface water resolution to ~1:100,000 scale based on a 30 m resolution national digital elevation model.
- NGIS: The National Groundwater Information System (NGIS) is a spatially-enabled groundwater database based on ArcHydro for Groundwater. NGIS has been populated with bore and bore log data (lithology, hydrostratigraphy and bore construction) from state and territory groundwater databases. NGIS also contains two- and three-dimensional aquifer geometry for some areas.
- NAF: The National Aquifer Framework (NAF) is a three-tiered system for naming and grouping geologic units, hydrogeologic units and hydrogeologic complexes in Australia. The NAF has been developed in conjunction with Geoscience Australia and groundwater agencies and is used to standardise state and territory terminology for aquifers in the NGIS.
- GDE Atlas: The National Atlas of Groundwater Dependent Ecosystems (GDE Atlas) presents the current knowledge of GDEs across Australia, and includes known GDEs as well as ecosystems that potentially use groundwater, divided into three broad classes: vegetation, surface water and subterranean ecosystems. It incorporates previous fieldwork, literature and mapped GDEs as well as potential GDEs based on interpreted remote sensing data. The GDE Atlas was developed by the National Water Commission with input from every state and territory and is hosted by the Bureau of Meteorology.
Well reports and geophysical logs are mostly held by state agencies. Open file reports are available, though digital copies of geophysical logs for older wells may need to be purchased from a commercial supplier.
Hydrogeological data are available through a number of state agencies (such as state geological surveys and state water departments); federal agencies (such as Geoscience Australia and ABARES); universities and private companies. In some states (such as Queensland) a significant amount of the groundwater monitoring data is collected from private bores and the licensing arrangements for using those data may be complex and prescriptive.
4.1.4.2 Maps
The BAs should use authoritative vector datasets maintained by federal and state agencies wherever possible. For example, the process of defining bioregions should use geological basin boundaries from the Geoscience Australia Geological Provinces database. Modifications and additions to these datasets should be fed back into the custodian agency.
4.2 Two- and three-dimensional representations of basins
Understanding the hydrogeology and the distribution of CSG resources within a bioregion will require, in addition to a thorough analysis of observations, the development of vertical cross-sections and three-dimensional representations of the geology and hydrogeology within the bioregion. Ideally, these representations would include three-dimensional distributions in space of coal-bearing sediments, aquifers and aquitards, as well as hydrodynamics. While these will initially be based on conceptual models (Section 2.5.2.3 and Section 4.3), where possible the representations should be quantitative, representing variations in important parameters in space to allow numerical modelling of groundwater behaviour in response to current and future development pathways (Section 2.5.2.5 and Section 4.4).
These three-dimensional representations will also form the basis of visualisation products to enhance interpretation and communication of results. Generating visualisation products is a discrete task that is required following development of two- and three-dimensional representations of the bioregion.
4.3 Conceptual modelling
A conceptual model is a qualitative description of the systems and sub-systems within a bioregion and a hypothesis or set of hypotheses as to how they interact with respect to impacts of CSG and coal mining development on these systems. It is a key component of the refinement of key aspects of the assessment's focus and is the primary mechanism by which the various contributors across disciplines develop a shared understanding of the assessment's goals. The conceptual models are closely linked with – and are used to aid the development of – the qualitative, semi-quantitative and quantitative models used to describe impacts on receptors (see Section 2.5.2.6). During the development of a BA, the conceptual model will undergo refinement as understanding improves. The conceptual model is the key device in the BA used to determine numerical model complexity, data requirements and uncertainty sources. It is also a key communications device on the state of scientific knowledge underpinning the BA.
In BAs, the conceptual models must define a causal pathway from depressurisation and dewatering of coal seams (event) to the direct, indirect and cumulative impacts on anthropogenic and ecological receptors (impact). They must emphasise the linkages between the key features of conceptualisation, numerical modelling, the system's water balance and impacts. The conceptual model also identifies key sources of uncertainty. All aspects of the conceptual models will be made fully transparent for external scrutiny. The conceptual models also have a role in explaining complex scientific processes to a broader community using two- and three-dimensional visualisation techniques.
Using the ecological, geological, hydrological and hydrogeological data, and the future development pathways (for CSG and coal resources), a conceptual model of the groundwater and surface water dynamics of a bioregion is to be constructed in accordance with the principles outlined in Section 2.5.2.3. The conceptual model begins with the geologic, seismic and stratigraphic data on vertical and lateral variation in rock types, faults and structure to identify potential compartmentalisation, cross-aquifer contamination risks and dewatering characteristics. It must define the main stratigraphic units and features; dominant mechanisms and locations of recharge, discharge and flows; surface water – groundwater interactions; and the location of CSG- and coal-associated impacts on surface or near-surface anthropogenic and ecological receptors taking into account the causal pathway of processes. This will set the design parameters for any numerical modelling, focus the objectives of the assessment, and guide the development of outputs towards assessment of impacts on receptors. As such, the conceptual model for any bioregion may comprise a comprehensive broad-scale model along with nested conceptual sub-models to take into account the range of time and space scales and connectivity over which processes occur. Many examples of conceptual models exist for different programs of research from which conceptual models for the BAs can be developed (e.g. groundwater modelling conceptual models in Barnett et al. (2012), conceptual models for surface water – groundwater interactions in Patterson et al. (2008), and the 'Healthy Waterways Program' ecological conceptual models for Southeast Queensland and the Queensland Wetlands Program). However, the conceptual model(s) developed in a BA are required to be more comprehensive and detailed to satisfy known characteristics and available data.
The conceptual model should capture important features of the physical properties of the groundwater and surface water systems within a bioregion and convey an understanding of spatial and temporal variation in those properties. It should:
- define the boundaries of the physical system under consideration
- summarise the major stratigraphic units, faults and other hydrogeologic features (such as distribution of hydraulic conductivity and porosity, trends in hydraulic head and flow characteristics, and assumptions about interconnectivity of strata)
- identify the dominant mechanisms and locations for recharge, discharge, flows and surface water – groundwater interactions
- identify the location of anthropogenic impacts on the surface water and groundwater hydrology
- identify uncertainties
- identify key questions that the assessment should answer.
One product of a BA is a well-defined conceptual model.
4.4 Surface water and groundwater numerical modelling
4.4.1 Flow model development
Modelling undertaken within a BA should adhere to recommended current best practice. Model complexity should be commensurate with data availability and complexity of the domain over which processes are to be simulated. However, it is expected that the complexity achieved in each BA will be at best practice such that groundwater and surface water responses to regional CSG and coal mining development and their impacts will be able to be assessed with an appropriate level of certainty.
A fully described, quantitative BA comprises a set of fully coupled groundwater, surface water and ecological impact models that directly link the dewatering of coal seams with impacts on receptors. These coupled models must also propagate statistical uncertainties associated with parameters and states through to uncertainties in impacts that can then be transformed to risk likelihoods. It is recognised that such a goal is difficult to achieve even in well-studied basins. However, these difficulties do not negate the requirement that a necessary condition for assessing impacts of coal seam dewatering on receptors is the linking of coal seam depressurisation effects on receptors via their water resource requirements. In most locations, such a high modelling capability will not be possible and more generalised modelling approaches will be required. In these cases, linkages between models may be indirect (i.e. not coupled) and rely on reasoning to establish links between component processes. The intent of the BA methodology is to specify an upper limit to what could be achieved in the best of circumstances. What is actually achieved in any particular BA may necessarily be of reduced scope and the BA methodology prescribes that all decisions are recorded in the workflow.
In light of the above, 'best-practice' BAs require a suite of tools and models covering groundwater and surface water dynamics, coupling of groundwater and surface water processes, and assessment of impacts. These should be capable of estimating quantitatively the uncertainties in state variables and parameters. Although fully quantitative models are desired, several different types might be suitable (empirical, qualitative, chemical speciation, statistical, etc.). Achieving 'best practice' in such a multidisciplinary environment requires:
- clear articulation of goals
- accurate definition of the state of knowledge
- appropriate use of models, data and calibration tools across a range of disciplines
- identification of uncertainties, assumptions and biases
- transparent recording of decisions
- successful marrying of information at different time and space scales from the water resource and petroleum industries
- an adaptive approach that iterates towards a final position within the time frame of the project.
For example, it may be necessary to resolve aquifer compartments within a bioregion undergoing coal seam dewatering in order to assess the risks of fault movement (and resultant connectivity) on an agricultural aquifer. It is recognised, however, that such a study would only be possible in locations where sufficient data and knowledge of model processes exist so that a comprehensive analysis of surface water and groundwater dynamics could be undertaken.
A detailed assessment of groundwater chemistry and isotopic composition for individual hydrostratigraphic units (including aquifers and coal seams) should be used where data are available to better understand the inter-aquifer connectivity in bioregions. This assessment should include analysis of naturally occurring hydrocarbon compounds present and their isotopic compositions in order to understand their likely origins and migration pathways before further major developments take place (Draper and Boreham, 2006). Appropriate data are unlikely to be available; therefore recommendations for a data collection program must be provided as part of the BA to establish the baseline groundwater chemistry information against which the impact of future activities may be measured. A comprehensive groundwater baseline survey would typically include field measurements, major and minor ions, trace elements, stable isotopes, organic compounds and dissolved gases. Since baseline data are essential for the detection of future changes, a baseline survey must take account of the spatial and temporal variation of groundwater chemistry and be undertaken in the context of a risk analysis and other risk assessments relating to the introduction of industrial chemicals.
A key component to the modelling is to explicitly link changes in hydrogeological characteristics of coal seams at depth and their adjacent aquifers and aquitards to those processes operating at the surface that impact on the receptors identified within key water-dependent assets. Without these explicit connections, a quantitative analysis of the direct impacts of dewatering will not be possible. Note that the explicit representation of 'causal pathways' is necessary to ensure that the receptor impact modelling (Section 2.5.2.6 and Section 4.5) is achieved.
From the conceptual model (Section 4.3), and the resulting specifications and objectives, the numerical model is to be constructed to an appropriate level of complexity. Groundwater modelling is to fully represent all relevant hydrostratigraphic units within the system and bioregion and their relevant interactions. A variable resolution model grid may be required where bioregions are complex and/or data are sparse.
Various industry and scientific models are available that have been designed for specific purposes and are able to perform components of a BA within a priority region (for example, models such as Petrel, Eclipse, RMS Roxar, MODFLOW or FEFLOW each explore different aspects of groundwater dynamics). Other models (either deterministic or statistical) deal with ecological function, thresholds and responses of receptors to forcing variables (for example threat–pathway–receptor models that explicitly link driver variables with impacts on receptors identified in the contextual information component). A BA will require synthesis of information (and uncertainties; Section 4.6) from a range of these models that operate on different time and space scales. As a result, it is important to define the extent and resolution of each model within a bioregion. For example, the following need to be commensurate with data availability, system knowledge and purpose:
- the spatial distribution and resolution of aquifer permeability
- vertical leakage rates between aquifers via fractures and faults
- pore space volumes
- hydraulic conductivity of aquitards and seals
- surface water flows and drainage
- receptor ecological responses (including vegetation and wetland data).
The appropriate methods for groundwater flow modelling, including calibration, prediction and uncertainty analysis, are provided in the Australian groundwater modelling guidelines by Middlemiss (2001) and Barnett et al. (2012) and these should be consulted as required.
Surface water modelling must represent spatially distributed rainfall-runoff processes, streamflow routing, evapotranspiration, deep drainage, and the impacts of climate variation on these flows. River models are to be used where required to assess impacts of changes in flow and recharge associated with CSG and coal mining development. These river models also need to address the potential influences of water management – such as flow diversions and infrastructure – on surface water hydrology. Where possible, the surface water models should be fully coupled to groundwater models. However, fully coupled models may not be possible due to the complexity involved in highly non-linear processes that are difficult to measure. A pragmatic solution might be to develop a suite of nested models and – based on explicit assumptions governing interactions between models at different scales – develop schemes for passing information between component models within the model suite. For example, nested surface water models may be required that use groundwater models as boundary conditions (e.g. Neumann boundary conditions for water fluxes). These boundary conditions provide constraints in addition to the usual observations of rainfall, evapotranspiration, runoff, offtakes, and storages in weirs and dams (see also Section 4.4.2 on calibration). Judgment will be required in determining the appropriate complexity and resolution of numerical models that maximise the advantages of simple models and utility of complex models. Decision points and action pathways associated with all aspects of modelling, calibration and simulation must be recorded in the workflow.
An assessment of the water balance needs to take into account surface water – groundwater interactions. The models need to simulate fluctuations in flow in rivers, connections with groundwater (including seasonal variation in baseflow), discharge to surface, flow through the unsaturated zone and deep drainage, and flows in saturated zones.
It should be noted that best-practice groundwater numerical modelling and impact analysis within useful uncertainty bounds will push the capability of current numerical models to their limit, even in well-studied locations. To achieve best practice, it is likely that significant model development will be required specifically on the topics of:
- using multiple observation types as multiple constraints on model dynamics
- improved representation of coupled surface water – groundwater processes in models
- implementation of computationally efficient methods to quantitatively estimate uncertainty.
Given differences in characteristic timescales of surface water and groundwater processes, it may be necessary to run the surface water and groundwater models on different time steps to ensure that the computation remains tractable. It will also be necessary to run groundwater and surface water systems to a 'dynamic equilibrium' (Rassam et al., 2008) and for sufficient time periods to fully realise impacts of dewatering.
In large, complex and data-sparse bioregions, this will likely necessitate a hierarchical approach to the numerical modelling of groundwater with variable resolution in time and space of processes across bioregions. For example, in high-priority bioregions, complex and highly resolved models may be required for important processes governing the dynamics of groundwater responding to development pathways of highly localised CSG and/or coal mining development. In low-priority bioregions that are relatively uniform areas away from CSG and/or coal mining developments, simpler models at coarser resolution may suffice. Irrespective of these requirements, the choice of model type may be dictated by data availability. Choices – as well as justification of these choices – need to be catalogued during model development in the workflow.
4.4.2 Calibration
Model calibration will need to use multiple lines of evidence and different types of data as constraints on the surface water and groundwater modelling to provide the most accurate numerical description possible of the current condition of water resources and the direct impact of water use across sectors including CSG and coal mining development.
Parameters and their uncertainties should be estimated using appropriate inverse methods and calibration techniques. Where possible, state estimation using data assimilation techniques may be required. Methods must be cognisant of the needs to quantify uncertainties in states, fluxes and parameters. Best-practice performance measures for model calibration should be used to explicitly quantify model performance and calibration against observations (including correlation, root mean square error, residual plots, etc.).
In the case of groundwater modelling, model scope, resolution, and calibration must use – where possible and appropriate – all available data as constraints on model dynamics, including constraints on boundary conditions, initial conditions, states and parameters. This includes, for example:
- geophysical data (TDEM, seismic, well log, gravity and electromagnetic) to better define boundary conditions and compartmentalisation of aquifers
- geochemical measurements (structural geology; fault characterisation; paleogeography; stratigraphy; noble gases; isotopes; tracers; and groundwater temperatures, pressures and chemistry) to estimate aquifer permeability and porosity.
Thus, calibration of groundwater models needs to focus on both geological and hydrological constraints. In some cases, these calibrated models may not provide a quantitative definitive prediction of coal seam behaviour under depressurisation; rather, they may supply information on significant impacts thereby underpinning an assessment based on multiple lines of evidence. Calibration methods must adopt, wherever possible, quantitative methods (i.e. state or parameter estimation and inverse methods) that are capable of:
- enabling quantitative propagation of statistical uncertainties
- communicating uncertainties in ways third parties can repeat results
- enabling assessment of future sampling and observation programs that maximally reduce uncertainties
- contributing to automation of updates to BAs as new data become available
- providing a means of integrating deterministic hydrologic models with the risk analysis
- In cases where insufficient data exist for model calibration given uncertainties, advice on impacts will be provided by the conceptual model(s) and through exploration of development pathways by bounding likely system responses. These models are also useful for designing field investigations to generate sufficient data for future BAs.
4.4.3 Prediction
The objectives of the numerical modelling are to:
- quantitatively assess the impacts of dewatering associated with CSG and coal mining development on aquifer properties, flows, pressure heads, the watertable, and chemical characteristics and transformations
- link depressurisation of coal seams (at depths of between a few tens of meters and less than 1000 m) to impacts on surface water dynamics, flows and groundwater-dependent systems
- reconcile multiple sources of observations on hydraulic characteristics, chemical constituents, pressure potentials and flows.
Output from the numerical modelling will be used to assess direct impacts on receptors (Section 2.5.3 and Section 5.2.1) given climate forcing and variation. This will also require a gap analysis to understand the significance of missing data and the development of ongoing sampling and monitoring programs for model improvement. Modelling time series must take into account the seasonal, inter-annual and inter-decadal climatic variability associated with regions within which CSG and coal mining development is taking place. In addition, while the time horizon for the development pathways may be decades, the modelling time horizon must be sufficiently long that model dynamics approach equilibrium within a specified tolerance. Thus, the time horizon for impacts may be centuries.
Output from the numerical simulations should be displayed using two- and three-dimensional visualisation tools as required for both model input and output data.
4.5 Receptor impact modelling
Modeling and analysis of the direct, indirect and cumulative impacts of CSG and coal mining development on anthropogenic and ecological receptors is the pivotal component of a BA. It is the point where all prior information along a causal pathway from depressurisation and dewatering of a coal seam to the surface impacts on receptors is 'synthesised' into conditional statements about the likelihood of impacts and the risks posed to values of water-dependent assets. For this reason, ecological knowledge acquired from a range of sources is required to provide advice on the resilience, resistance, vulnerability and response of ecological receptors (and hence assets). Anthropogenic receptors may require ecological or additional information to undertake impact modelling. The receptor impact modelling is closely connected with the impact analysis (Section 5.2).
4.5.1 Model development
Receptor impact modelling will be required for each identified receptor or group of receptors. Receptors may indicate the condition and trend of several assets, or several receptors may be conceptually expected to respond similarly to changes in water quality or quantity. As a result, aggregation of receptors may be required, particularly if it is necessary to ensure manageable amount of work in the BA given time constraints.
Responses of anthropogenic and ecological receptors to dewatering of coal seams will depend on the distance, stratigraphic proximity, connecting pathways, resistance, resilience, response, and threshold condition of a receptor to the forcing perturbation (i.e. dewatering). An assessment of receptor exposure is necessary to determine whether impacts are chronic or acute, whereas receptor resilience determines whether receptor state will return to pre-perturbation condition following the dewatering event. An assessment of threshold levels may also be required if there is potential for chronic exposure or significant perturbation to lead to impacts that change receptor baseline state or condition. The impact modelling will need to consider relationships between environmental driver variables and changes in response variables in receptors.
Anthropogenic receptors (e.g. agricultural wells) in many cases will potentially experience direct impacts of dewatering from CSG and coal mining development (e.g. loss of hydraulic head) and, in circumstances where multiple developments exist, these direct impacts will be cumulative. Ecological receptors (e.g. a rare and threatened freshwater species within a wetland) will potentially experience indirect impacts (e.g. loss of flow arising from a decrease in hydraulic head in a groundwater-connected surface wetland). The degree of impacts on receptors will depend on the distance from dewatering, vertical stratigraphic proximity to dewatering, connecting pathways between depressurisation and dewatering and receptors, as well as the resistance, resilience, response and threshold condition of receptors to dewatering.
Receptor impact model development should consider:
- clear articulation of the aim of the model
- baseline data providing current status
- identification of uncertainties, assumptions and biases in identifying presumed magnitude and direction of change.
Depending on the level of understanding of the type, magnitude and direction of change that impacts may have on receptors, data from conceptual, semi-quantitative or quantitative numerical models will need to be incorporated. The levels of confidence around each of these must be recorded. Given existing data and modelling knowledge, it will be a significant challenge to quantitatively relate direct impacts of coal seam depressurisation and dewatering to groundwater and surface water flows and then subsequently (via a causal pathway) to impacts on receptors. Judgment as to the type of models and their application lies with individual researchers undertaking assessments and so it is not possible to be prescriptive as to the detailed methods to be applied. Nevertheless, all decisions based on researcher judgment must be recorded in the workflow and are subject to scrutiny by peer review as part of the process to ensure project transparency. As such, 'best practice' in this context constitutes balancing the complexity of receptor impact models with available data and knowledge, taking into consideration confidence in the data.
In some instances, particularly for ecological assets, it may prove impossible to adequately model receptors due to lack of understanding of mechanisms involved, need for potentially invasive techniques to monitor, or insufficient baseline data. In such instances, appropriate surrogates may be nominated which reflect the impact in a manner more amenable to monitoring. All inferences associated with the identification of surrogates should also be recorded.
4.5.2 Prediction
The objective of receptor impact modelling is to provide a robust framework within which to assess the potential exposure of assets through understanding the magnitude, direction and nature of changes effected on nominated receptors. The models need to be sufficiently clearly documented to allow reappraisal of impact in light of new or improved numerical modelling or other pertinent data, to contribute to understanding and monitoring of cumulative impacts, and to identify the means by which remediation or avoidance approaches may act.
Output from receptor impact modelling should be displayed using two- and three-dimensional visualisation tools as required for both model input and output data.
4.6 Uncertainty analysis
The BA methodology requires that uncertainties in observations and modelling be propagated to model output and expressed in quantitative terms wherever possible. The workflow should capture decisions made as to the level of sophistication of methods used, data availability and expression of forms of uncertainties.
The quantification of uncertainties is fundamental to many aspects of the BA methodology:
- the analysis of direct, indirect and cumulative impacts
- risk analysis
- design of future sampling programs to ensure new data maximally reduce uncertainties in modelling (e.g. Dausman et al., 2010).
Any qualitative or semi-quantitative methodology for risk analysis must ensure transparency and logical consistency in its consideration of likelihoods and consequences. Transparency means that qualitative descriptors are defined unambiguously and in a way that can be assessed and tested against data either now or in the future. This transparency will ensure that the decision taken will be based on common assumptions and understanding. Logical consistency will enable risk rating matrices to be constructed in a rigorous way. Best practice in this context refers to analytical or numerical methods used for quantifying likelihoods of parameters, measures of bias in model inputs and outputs, and characterisation of goodness-of-fit of model states to observations. Other sources of uncertainty arise from imprecision, ambiguity, vagueness and lack of clarity; these must also be considered.
Other significant sources of error must be incorporated into analyses – for example, the effects of non-steady state conditions when a steady state assumption has been used in groundwater model calibration or biases introduced through linearisation methods. Accurate baseline conditions and human-induced impacts of CSG and coal mining development must be determined as best as possible. This is important in distinguishing change due to, for example, climate variation from causal impacts due to dewatering for coal resource extraction. A key issue will be the separation of background Earth processes (dominated by geology) from processes triggered by dewatering and/or drilling practices. In many cases, it will be difficult to obtain definitive data to distinguish 'natural' impacts from impacts from coal resource development. Under these circumstances, impacts need to be described in terms of multiple lines of evidence. Bioregional analyses must identify, for example:
- conceptual model uncertainty: epistemic uncertainty or imprecision (which represents an absence of knowledge). This could, for example, include the uncertainties associated with potential connectivity and flow systems between aquifers, fault movement and seal leakage, and lateral distribution of seams and aquitards
- parameter uncertainty or random effects: uncertainties associated with aquifer transmissivity or porosity over space derived from random errors in observations using model calibration methods
- development pathway uncertainty: the uncertainty associated with CSG and coal mining development must be assessed and incorporated in the development pathways used in the impact analysis
- model and data biases: the source and quantity of bias arising from datasets and models must be assessed and bias correction procedures implemented where possible.
These sources of uncertainty provide a basis for impact analysis using analytic or numerical methods for the propagation of uncertainties in models as appropriate. For example, the Monte Carlo method coupled with Latin hypercube sampling is a computationally efficient approach to developing co-variances among model driver variables that are needed to generate likelihoods of output variables. Stochastic realisations of models based on these co-variances (or expected variations in driver variables and parameters in the absence of other information) is the best available means of determining model behaviour arising from uncertainty in parameters such as base and peak flows of streams, and porosity, hydraulic conductivity and storativity of aquifers. In some cases, coupling of simple and complex models will provide a means to estimate and remove bias while addressing issues of data availability and assessing impacts on receptors at relatively fine scales (Doherty and Christensen, 2011). Data paucity is not necessarily a justification for application of simple models if those models do not address impacts on the receptors identified in the contextual information component (Hunt et al., 2007). In order to estimate impacts and their uncertainties within localised regions, it may be necessary to use more complex models, or, for example, nested models with variable resolution in locations of interest.
A checklist of sources of uncertainty must be developed and used as a basis for developing the impact analysis. A checklist will include, but not be limited to, uncertainties in the following:
- boundary conditions
- driver data
- model parameters
- conceptual model structure
- discretisation
- scale transitions
- computation and numerical routines.
It is recognised that developing robust quantitative estimates of model uncertainties will be difficult given available data and limited knowledge in some bioregions. In data-sparse bioregions, and in the absence of a fully quantitative analysis of probabilities of model outputs, a sensitivity analysis should accompany numerical modelling in order to support semi-quantitative or qualitative estimates of uncertainty.
Model predictions that result from assuming a given future development pathway should provide means for testing and validation, recognising both the uncertainties in model output and that the development of groundwater responses to depressurisation may take several decades.
Practitioners undertaking BAs will need to decide:
- the appropriate time and space scales to focus on
- how outputs from numerical groundwater models will be translated to surface water models
- how to express ecological impacts via direct links or use of metrics or surrogate variables in terms that can be readily understood by a wide audience (Hancock et al., 2009).
The estimates of uncertainty need to be clearly expressed in a manner that is understandable to an outside audience and decision makers. Engagement with external agencies and stakeholders must occur early in the project during the assembly of contextual information to determine suitable representations of uncertainties and levels in confidence in models. In the case of coupled models (for example, coupled surface water and groundwater models), suitable schemes for transferring uncertainties between scales need to be devised and recorded in the workflow. The transfer of information between models must take into account variation in the time and space scales associated with each model. For example, decadal processes in a groundwater model may be represented as slowly evolving boundary conditions in an ecological impact model. In turn, daily processes within a surface water model may be 'seen' by a groundwater model as a mean with stochastic variation.
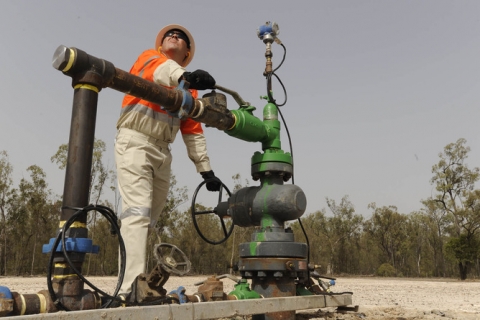