- Home
- Assessments
- Bioregional Assessment Program
- Clarence-Moreton bioregion
- 2.3 Conceptual modelling for the Clarence-Moreton bioregion
- 2.3.2 Summary of key system components, processes and interactions
- 2.3.2.4 Hydraulic connection between aquifers and surface water and groundwater
2.3.2.4.1 Aquifer interaction
As shown in previous sections, there is a high degree of hydraulic connection between the alluvial and volcanic aquifers in the Clarence-Moreton bioregion with the greatest level of interaction in the headwaters of the alluvial systems, decreasing down-gradient.
The hydraulic relationships between different sedimentary bedrock units, sedimentary bedrock and alluvial aquifers and between sedimentary bedrock and volcanic bedrock are much more difficult to determine than the interaction between shallow aquifers. This is due to the complexity of the Clarence-Moreton Basin aquifer/aquitard system and the lack of groundwater observation bore data for the sedimentary bedrock units. The current conceptual model for the Main Range Volcanics in Queensland (Figure 23) assumes that there is only limited vertical connectivity across the interface between the basalts and the underlying sedimentary bedrock due to the presence of weathering horizons with very low hydraulic conductivities. Some downward percolation of groundwater probably occurs across the interface of the basalt and the underlying sedimentary bedrock, as supported by hydrochemical data in the Logan-Albert river basin within the Clarence-Moreton bioregion (Duvert et al., 2015). The lack of groundwater observation bore data in the Richmond river basin means that hydraulic head gradients between the volcanic and sedimentary bedrock units are poorly constrained. However, the elevated topography of the basalts and the substantially higher recharge rates suggest that there is more likely to be a downward hydraulic gradient from the basalts to the underlying sedimentary bedrock in most areas. Despite some evidence for hydraulic connectivity across this interface (Duvert et al., 2015), the role of downward percolation from the basalts to the underlying sedimentary bedrock is thought to be small in most areas as a result of the presence of thick weathering profiles developed on the sedimentary bedrock prior to eruption of the volcanics that limit vertical leakage across this interface due to their low hydraulic conductivities (Figure 23).
The lack of nested bore sites within the Clarence-Moreton bioregion that enable different sedimentary bedrock stratigraphic units to be simultaneously monitored significantly limits the understanding of inter-aquifer connectivity as it is currently based primarily on inferred petrophysical characteristics of different stratigraphic units and on aquifer geometry. Most sedimentary bedrock units in the Clarence-Moreton bioregion are considered as partial aquifers, aquitards or leaky aquitards (Section 2.3.2.2.7; Figure 26). If an aquitard is an ideal regional seal, then it should limit or prevent the vertical hydraulic connection between the overlying and underlying stratigraphic units. In the case of the Walloon Coal Measures of the central part of the Richmond river basin, Doig and Stanmore (2012) suggested that high gas saturation levels are an indicator that there is an effective seal in place in the central part of the Richmond river basin near the West Casino Gas Project that prevents or limits vertical gas leakage from the Walloon Coal Measures. This seal is assumed to be the Maclean Sandstone, which is between the coal seams of the Walloon Coal Measures and the Kangaroo Creek Sandstone Member. In the West Casino Gas Project area west and south of Casino (Raiber et al., 2015), the Walloon Coal Measures are approximately 300 to 600 m below ground surface, and in addition to the Maclean Sandstone, there are two other stratigraphic units that are considered as aquitards (Bungawalbin Member and Rapville Member) separating the coal seams of the Walloon Coal Measures and the shallow alluvial and volcanic aquifers. Although aquitards are spatially and stratigraphically continuous throughout the West Casino Gas Project area west and south of Casino, their thickness and composition can vary. Furthermore, their role as regional seals may be compromised by the presence of geological structures (i.e. faults, which can act either as conduits or barriers to groundwater flow) or regional differences in rock composition. There are several major geological structures within the Richmond river basin (e.g. East Richmond and Coraki faults; Figure 8) where fault displacement is known to occur. High groundwater salinities in the alluvium near Coraki (Figure 18) and the similarity between the course of Myrtle Creek and the orientation of Coraki Fault could be an indication that this fault acts as a conduit for upwards discharge of deep groundwater to shallow aquifers or to the surface. However, further work throughout the Richmond river basin (e.g. use of remote sensing to determine which major faults have a surface expression, environmental tracer sampling and determination of shale gouge ratios and juxtaposition values of aquifers and aquitards) and supporting data are required to assess the role of faults on the groundwater flow system. There are currently no field data (e.g. groundwater level measurements from multi-level monitoring bores) available to assess the role of faulting on groundwater systems in the Clarence-Moreton bioregion.
The hydraulic connection between the sedimentary bedrock and the alluvial aquifers is thought to be much less pronounced compared to that between the volcanic and alluvial aquifers, due mostly to the low permeability of the sedimentary bedrock (Section 2.3.2.2.7). However, the degree of hydraulic connectivity is variable both spatially and temporally. For example, increasing groundwater salinity during droughts in parts of the Lockyer Valley alluvial aquifers is linked to leakage from the underlying sedimentary bedrock (Gatton Sandstone), which contains more-saline water (McJannet et al., 2015; Raiber et al., 2015). In the central Richmond river basin east and south of Casino, elevated groundwater salinity in some groundwater bores screened in the alluvial aquifer suggests some degree of hydraulic connectivity between the sedimentary bedrock and the shallow alluvial aquifers (McJannet et al., 2015; Raiber et al., 2015). However, the recharge assessment (Figure 12) has shown that recharge rates in the alluvial aquifer in these areas are lower than elsewhere within the catchment due to the presence of thick clay-rich floodplain sediments, and the elevated groundwater salinities could therefore also be the result of enhanced levels of evapotranspiration.
In the eastern part of the Clarence-Moreton bioregion where different sedimentary bedrock stratigraphic units thin and pinch out at the basin margin or subcrop underneath the alluvial aquifers (Figure 24), upwelling of groundwater from the sedimentary bedrock into the shallow alluvial aquifers is likely. Although the flow directions in the sedimentary bedrock of the Richmond river basin are poorly constrained by monitoring data, an example from the Bremer river basin (which is well supported by monitoring data) shows how groundwater in the sedimentary bedrock units flows from the ranges towards the lowest point of the catchment, where it is likely to discharge into the Bremer River west of Ipswich (Raiber et al., 2015). The analogue example of the Bremer river basin suggests that groundwater discharges from the sedimentary bedrock to the alluvium at the down-gradient end of the Richmond river basin. However, while there are some elevated salinities in the alluvium that may indicate upward discharge of more-saline sedimentary bedrock groundwater, overall, most alluvial groundwaters in areas where the alluvium overlies the down-gradient end of the sedimentary bedrock are fresh (Figure 18). This suggests that upwards leakage from the sedimentary bedrock may be overwhelmed by other sources of recharge to the alluvium, such as river recharge or diffuse rainfall recharge through soils, which become increasingly sandy near the coast.
2.3.2.4.2 Surface water – groundwater interactions
Throughout the Richmond river basin and other areas within the Clarence-Moreton bioregion, there is very strong evidence for interaction between groundwater and surface water. The estimation of groundwater recharge rates and streamflow volumes highlights that the geological unit with the main influence on the hydrological cycle in the Richmond river basin is the Lamington Volcanics. The basalts are recharged during rainfall events and water is stored and transmitted through vesicles and fracture zones. Some of the recharged water in the high energy upper or mid-reaches of the river system drains with very short lag times (near instantaneous to days) to the alluvium or directly to surface water or springs where no alluvium is developed following short flow paths. However, even after extended periods of no rainfall (and thus, no recharge), groundwater continues to drain from the basalts following longer flow paths. This continuous baseflow from the basalts generates the high median flow observed in the upper and mid-reaches of the Richmond river basin, and sustains the permanency of streams even during prolonged periods of no rainfall (Figure 27).
In the mid and lower reaches of the Richmond river basin and other catchments within the Clarence-Moreton bioregion, the hydraulic gradient between alluvial aquifers and streams can reverse during different climatic periods (Atkins et al., 2016). An example from the Lockyer Valley (Figure 20) shows how the deeply incised Laidley Creek changes from a disconnected creek at the climax of a prolonged drought in 2007 to a losing stream (where the stream recharges the underlying alluvial aquifer) in 2009 following the break of the drought and into a gaining stream in 2013 (where baseflow from the alluvia to the streams occurs). This complex temporal pattern of surface water – groundwater interaction, likely to be similar in mid-reaches of other streams in the Clarence-Moreton bioregion, highlights the challenges in defining whether river reaches are gaining or losing, and the need for long-term time-series groundwater-monitoring data. However, unlike Laidley Creek in this mid-reach location in the Lockyer Valley, digital elevation models suggest that the Richmond River and smaller streams in the Richmond river basin are not deeply incised into the alluvial aquifer. Furthermore, lithological logs from groundwater bores show that the alluvium in the mid to lower reaches of the Richmond river basin consists of thick clay-rich sediments at the top of the sequence. Streams have probably not fully penetrated these clay-rich sediments and therefore there may only be limited hydraulic connection with the more permeable sands and gravel at the base of the alluvial sequence. Consequently, surface water recharge to the alluvial aquifer may be limited, and there are likely to be considerable lag times between rainfall and recharge to the alluvial aquifer. Examples from the Clarence-Moreton bioregion in Queensland indicate large time lags between rainfall and groundwater level response (e.g. Figure 22).
The assessment of median streamflow rates (Section 2.3.2.2) shows that smaller streams such as Shannon Brook and Myrtle Creek in the central or southern part of the Richmond river basin have much smaller flow rates than the Richmond River and other streams draining the volcanics (Figure 27). There is likely to be a baseflow contribution from underlying aquifers to these streams, but unlike many of the streams that drain the Lamington Volcanics, these smaller streams that drain the sedimentary bedrock units rather than the Lamington Volcanics are not permanent.
One key aspect of surface water hydrology in the Richmond river basin is that the lower reaches of many streams show tidal influence. For example, the Richmond River is tidal to almost Casino. Elevated groundwater salinities were observed in shallow bores near Coraki (Atkins et al., 2015; Raiber et al., 2015), but it is currently not clear whether this is due to leakage from tidal rivers, slow infiltration promoting high rates of evapotranspiration prior to recharge or upwards discharge of more-saline sedimentary bedrock groundwater. The origin of this more-saline groundwater could likely be determined using additional hydrochemical data (major and minor ions) and selected environmental isotopes.
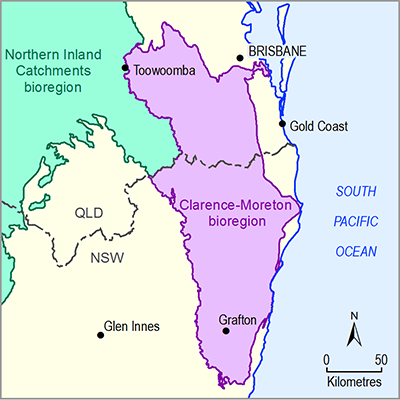