- Home
- Assessments
- Bioregional Assessment Program
- Hunter subregion
- 3-4 Impact and risk analysis for the Hunter subregion
- 3.4 Impacts on and risks to landscape classes
- 3.4.4 'Groundwater-dependent ecosystem (GDE)' landscape group
3.4.4.1 Description
GDEs are those ecosystems that rely on the surface or subsurface expression of groundwater to meet all or some of their life-cycle requirements (Eamus et al., 2006). The dependence of GDEs on groundwater varies both spatially and temporally (Eamus et al., 2006). Ecosystems may be obligate GDEs, with a continuous or entire dependence on groundwater, or facultative GDEs, with an infrequent or partial dependence on groundwater (Zencich et al., 2002). Plants that depend solely on moisture held within the soil profile are known as vadophytes and are not groundwater dependent (Sommer and Froend, 2010). In the Hunter subregion, as in much of Australia, there is considerable uncertainty as to the nature of groundwater dependency for much terrestrial vegetation.
The water requirements of GDEs are poorly understood and there is large uncertainty as to the frequency, timing and duration of their groundwater use (Andersen et al., 2016). In general, transpiration of groundwater is expected to decline as the depth to groundwater increases, but there is very limited evidence to support this assumption within Australia. O’Grady et al. (2010) reviewed estimates of groundwater discharge in Australia and concluded that there is considerable variation in the relationship between transpiration of groundwater and depth to groundwater. Factors such as the rooting depth of a particular species (which is usually not known), hydroclimatic environment and groundwater salinity all impact on groundwater use by vegetation. Zolfaghar et al. (2014) examined the structure and productivity of eucalypt forest across a depth to watertable gradient in the Upper Nepean catchment in NSW. They found that where groundwater was shallow, vegetation had significantly higher biomass and productivity than sites where groundwater was deeper than approximately 10 m. The relationships between depth to groundwater and the structural and functional attributes of the vegetation communities were highly non-linear, with steep declines in leaf area index and biomass over a range of 5 to 10 m depth to groundwater. However, it is important to note that the study was largely correlative in nature and did not quantify the groundwater requirements of the vegetation. Specific studies of GDEs within the Hunter subregion are limited. Existing mapping of GDEs is based on a multiple-lines-of-evidence approach that incorporated existing vegetation mapping, modelled groundwater levels and remote sensing (Kuginis et al., 2016). Modelled depths to groundwater (Summerell and Mitchell, 2011) for the subregion are generally shallow (within 16 m of the ground surface). However, there is likely to be uncertainty in the mapping owing to the sparse network of monitoring bores over much of the subregion.
The hydroclimatic environment of the Hunter subregion is subtropical in the eastern part, and bordering on temperate in the western part of the subregion. Average annual rainfall ranges from 600 to 1440 mm/year, with higher values associated with higher elevations and coastal areas. Precipitation is summer dominated when potential evaporation is also highest (see companion product 1.1 for the Hunter subregion (McVicar et al., 2015)). Hence, the region is classified as being water limited in as much as potential evaporation (1250 to 1950 mm/year) exceeds rainfall in most months of the year. In areas that experience a rainfall deficit, vegetation may be dependent to varying extents on groundwater within the Hunter subregion.
The geomorphology and hydrogeology of the Hunter subregion is described in companion product 1.1 (McVicar et al., 2015) and only a brief summary is presented here as context. The hydrogeological systems in the Hunter subregion are associated with Permian-Triassic rock aquifers, alluvial aquifers along major rivers and creeks and aeolian sands aquifers in the coastal zone of the subregion. The Hunter Valley represents a regional groundwater discharge zone and a dividing streamline for groundwater flow. The main regional surface water – groundwater fluxes largely follow the subregion topography from the upland towards the river channels with overall discharge towards the Tasman Sea. From a surface water perspective, the Hunter subregion is primarily composed of the Hunter river basin (87.5% of the subregion) and the Macquarie-Tuggerah lakes basin (10.7% of the subregion) (see companion product 1.1 for the Hunter subregion (McVicar et al., 2015)).
The subregion has three main hydrogeological units (see companion product 1.1 for the Hunter subregion (McVicar et al., 2015)) relevant to sustaining GDE structure and function, which provide a useful conceptual framework for examining landscape classes that are dependent on groundwater:
- alluvial aquifers along major rivers and creek lines
- fractured rock aquifers of the Hunter subregion
- coastal aquifers in the coastal area.
3.4.4.1.1 Alluvial aquifers
Alluvial aquifers form in sediments such as gravel, sand, silt and/or clay deposited by physical processes in river channels or on floodplains (Figure 47). These unconsolidated sedimentary aquifers may be layered and/or discontinuous due to the presence of deposits of low permeability silt and clay within the alluvia (Queensland Government, 2013a). Alluvial aquifers are generally shallower than sedimentary and fractured rock aquifers and water levels often fluctuate due to varying recharge and pumping rates (Geoscience Australia, 2016). Alluvia may be underlain by impermeable layers, which separate the unconfined sedimentary aquifer from other groundwater aquifers. Alluvia may support a range of ecosystems (Queensland Government, 2013a). Palustrine (e.g. swamps) and lacustrine (e.g. lakes) wetlands and riverine (e.g. streams and rivers) water bodies on alluvial deposits may depend on the surface expression of groundwater, while terrestrial vegetation may depend on subsurface groundwater that is typically accessed through the capillary zone above the watertable. Unconsolidated sedimentary aquifers in alluvial deposits may also support ecosystems within the aquifer itself, such as stygofauna.
The alluvial aquifer of the Hunter subregion is considered a regional discharge zone for the aquifers within the region (EPA, 2013), implying an interaction from the groundwater to surface water system through the alluvial aquifer. The connection between the alluvial aquifer and underlying fractured rocks is considered bi-directional (EPA, 2013). Groundwater discharge contributes to baseflow throughout the subregion, but is more persistent in the main Hunter alluvial systems than in the elevated areas, where there is a permanent connection to groundwater. The main recharge mechanisms for the alluvial aquifer are river leakage to the alluvium (particularly during flooding), direct rainfall recharge and upward flow from Permian fractured rocks (see companion product 1.1 for the Hunter subregion (McVicar et al., 2015)). River leakage is generally considered to be the largest recharge component, and in various modelling studies it has been fitted as up to four times greater than diffuse rainfall recharge (Worley Parsons, 2009; Heritage Computing, 2012).
GDE landscape classes, which are based on vegetation forms from Keith (2004) that are likely to be associated with alluvial aquifers, include forested wetlands, and some freshwater wetlands, rainforests and semi-arid wetlands.
Source: adapted from Queensland Government (2013a); Queensland Department of Science, Information Technology and Innovation (Dataset 8)
© The State of Queensland (Department of Environment and Heritage Protection) 2012–2017
3.4.4.1.2 Fractured rock
In fractured rock aquifers (Figure 48), groundwater is stored in the fractures, joints, bedding planes and cavities of the rock mass (Geoscience Australia, 2016) and transmitted through fractures within the otherwise low-permeability rock (Queensland Government, 2015). Fractured rock aquifers may discharge groundwater into channels largely in the lower parts of the landscape while channels in upper parts of the landscape usually transmit surface water runoff only. Groundwater diffuse recharge from Permian sedimentary rock units in the Hunter subregion is estimated at less than 2% of annual rainfall, with high values associated with areas of the enhanced regolith permeability.
GDE landscape classes most likely to be associated with fractured rock aquifers include some rainforests, wet and dry sclerophyll forests, grassy woodlands and semi-arid woodlands.
Figure 48 Conceptual model of the major groundwater processes in fractured rock systems
The number ‘1’ indicates a surface expression groundwater-dependent ecosystem (GDE).
Source: Queensland Government (2015); Queensland Department of Science, Information Technology and Innovation (Dataset 8)
© The State of Queensland (Department of Environment and Heritage Protection) 2012–2017
3.4.4.1.3 Coastal aquifers
Coastal sands typically support a single, unconsolidated sedimentary aquifer, in which groundwater forms a freshwater lens in the intergranular voids of the coastal sand mass (Figure 49). Perched aquifers may also occur over low permeability layers within the sand mass (Queensland Government, 2013b). Palustrine (e.g. swamps) and lacustrine (e.g. lakes) wetlands and riverine (e.g. streams and rivers) water bodies on coastal sand masses may depend on the surface expression of groundwater from these unconsolidated sedimentary aquifers, while terrestrial vegetation may depend on subsurface groundwater that is typically accessed through the capillary zone above the watertable. Unconsolidated sedimentary aquifers may also support subterranean ecosystems within the aquifer itself, indicated by the presence of stygofauna.
In the Hunter subregion, dunal and/or coastal (of aeolian origin) sands of medium-grain size form a highly permeable unconfined aquifer, and rainfall rapidly infiltrates through the unsaturated zone to recharge the saturated zone (companion product 1.1 for the Hunter subregion (McVicar et al., 2015)). This type of aquifer experiences the highest diffuse recharge rate in the subregion. Transmissivity was estimated between 400 m/day to more than 600 m/day (Crosbie, 2003), with a specific yield of about 0.2. The groundwater level is very responsive to rainfall events, with groundwater level rises over a metre observed on an event basis (Crosbie, 2003).
GDE landscape classes most likely to be associated with coastal aquifers include some freshwater wetlands, forested wetlands, heathlands and rainforests. Estuarine and near-shore marine ecosystems located adjacent to coastal sand masses may also depend on the discharge of groundwater from these unconsolidated sedimentary aquifers (note that ‘Coastal lakes and estuarine systems’ are covered in Section 2.7.5 in companion product 2.7 for the Hunter subregion (Hosack et al., 2018a)).
Figure 49 Conceptual model of the major groundwater processes on coastal areas
Numbers refer to types of groundwater-dependent ecosystems (GDEs) as follows: 1 = terrestrial GDEs, 2 = surface expression GDEs, 3 = subterranean GDEs, 4 = surface expression GDEs (estuarine systems), 5 = surface expression GDEs (near-shore marine systems).
Source: Queensland Government (2013b); Queensland Department of Science, Information Technology and Innovation (Dataset 8)
© The State of Queensland (Department of Environment and Heritage Protection) 2012–2017
3.4.4.2 Potential hydrological changes
The area of GDE landscape classes within the zone of potential hydrological change is 102 km2 of which 1.8 km2 is in the mine pit exclusion zone (Table 20). This represents 28% of the total area of GDEs within the Hunter assessment extent (Table 20). Only small percentages (<3%) of the total areas of ‘Freshwater wetland’, ‘Grassy woodland’, ‘Heathland’ and ‘Semi-arid woodland’ are present in the zone. Instead, 16% of ‘Dry sclerophyll forest’, 38% of ‘Forested wetland’, 60% of ‘Rainforest’ and 31% of ‘Wet sclerophyll forest’ landscape classes are present in the zone (Table 20).
All the ‘Rainforest’ (24 km2) and ‘Wet sclerophyll forest’ (4.5 km2) in the zone are present in the Macquarie-Tuggerah lakes basin, as is the majority of the ‘Dry sclerophyll forest’ (13.2 km2); small areas of ‘Dry sclerophyll forest’ (1.2 km2) are present in the Upper Goulburn. Forested wetlands are most concentrated in the Macquarie-Tuggerah (28.8 km2) and Central Hunter (13.2 km2), with smaller areas in the Lower Goulburn (9.2 km2), Lower Hunter (4.7 km2) and Upper Goulburn (1.9 km2).
The hydrological factors identified by experts in the qualitative modelling workshops (see companion product 2.7 for the Hunter subregion (Hosack et al., 2018a)) have been interpreted as a set of hydrological response variables. The hydrological factors and associated hydrological response variables for the ‘GDE’ landscape group are the:
- maximum difference in drawdown under the baseline future or under the coal resource development pathway future relative to the reference period (1983 to 2012) (dmaxRef)
- year that the maximum decrease of groundwater occurs (tmaxRef)
- mean annual number of events with a peak daily flow exceeding the volume of flow that is assumed to result in ‘overbench’ flow (EventsR0.3)
- mean annual number of events with a peak daily flow exceeding the volume of flow that is assumed to result in ‘overbank’ flow (EventsR3.0).
For details of these variables see Section 2.7.3 in companion product 2.7 for the Hunter subregion (Hosack et al., 2018a). Drawdown is a hydrological response variable for both the ‘Forested wetland’ and ‘Wet and dry sclerophyll forest’ receptor impact models, while the overbench and overbank flow hydrological response variables are only used in the ‘Forested wetland’ receptor impact model.
3.4.4.2.1 Groundwater
Of the 100 km2 of GDEs outside the mine pit exclusion zone, 39 km2 are potentially subject to drawdown of more than 0.2 m under the baseline (95th percentile); this reduces to 14 km2 at the 50th percentile and 7 km2 at the 5th percentile (Table 20). The majority of the GDE area potentially subject to drawdown under the baseline future is ‘Forested wetland’, ‘Rainforest’ or ‘Dry sclerophyll forest’ (Figure 50). Approximately half of the ‘Forested wetland’ is riverine forest (29.1 km2) and half is coastal forest in the Macquarie-Tuggerah lakes basin (28.7 km2). For the majority of the GDE area predicted to experience drawdown under the baseline drawdown, the predicted drawdown is less than 2 m. For GDEs, 9 km2 will potentially experience a drawdown of more than 2 m under the baseline, most of which is ‘Forested wetland’ or ‘Dry sclerophyll forest’.
As a result of additional coal resource development, 62 km2 of GDEs are potentially subject to additional drawdown of at least 0.2 m (95th percentile); this reduces to 41 km2 at the 50th percentile and 16 km2 at the 5th percentile (Table 21; Figure 51). The majority of the GDE area potentially subject to additional drawdown is ‘Forested wetland’, ‘Rainforest’ or ‘Dry sclerophyll forest’. Twenty-eight km2 of GDEs are predicted to experience an additional drawdown of more than 2 m and 17 km2 are potentially subject to additional drawdown of greater than 5 m. ‘Rainforest’ is potentially subject to greater drawdowns than either ‘Forested wetland’ or ‘Dry sclerophyll forest’.
The vast majority of ‘Wet sclerophyll forest’, ‘Dry sclerophyll forest’ and ‘Rainforest’ landscape classes potentially subject to 0.2 m of drawdown due to additional coal resource development is in the Macquarie-Tuggerah reporting area (Figure 51); 5.6 km2 of ‘Wet sclerophyll forest’ or ‘Dry sclerophyll forest’ are potentially subject to drawdown of more than 2 m.
Nearly all of the riverine ‘Forested wetland’ in the Hunter and Goulburn river basins is potentially subject to drawdown of less than 2 m (median estimate); 2.6 km2 of the coastal ‘Forested wetland’ are potentially subject to drawdown of more than 2 m (median estimate).
When local geological and hydrogeological data are used to constrain model results based on the regional parameter set, the area potentially at risk of drawdowns exceeding 0.2 m can be smaller than predicted using the full set of simulations. This is illustrated for the Wyong River catchment in Figure 47 in companion product 2.6.2 for the Hunter subregion (Herron et al., 2018), and in Table 10 of this product. The areas of the potentially impacted GDE landscape classes in the Wyong River catchment based on the regional and local parameter sets are provided in Table 22 and Table 23. Using the locally-constrained parameter set, the area of GDEs identified as potentially at risk (at least 5% chance of greater than 0.2 m drawdown) due to additional coal resource development is just over 0.6 km2, about half the area (1.18 km2) estimated using the regional parameter result set. The biggest reductions in potentially impacted area are for the ‘Forested wetland’ GDEs, followed by ‘Rainforest’ GDEs.
Additional drawdown is the maximum difference in drawdown (dmax) between the coal resource development pathway (CRDP) and baseline, due to additional coal resource development.
Data: Bioregional Assessment Programme (Dataset 1, Dataset 3, Dataset 4, Dataset 5)
Additional drawdown is the maximum difference in drawdown (dmax) between the coal resource development pathway (CRDP) and baseline, due to additional coal resource development.
Data: Bioregional Assessment Programme (Dataset 1, Dataset 3, Dataset 4, Dataset 5)
Table 20 Area (km2) of landscape classes in the ‘Groundwater-dependent ecosystem (GDE)’ landscape group potentially exposed to varying levels of baseline drawdown in the zone of potential hydrological change
The area potentially exposed to ≥0.2, ≥2 and ≥5 m baseline drawdown is shown for the 5th, 50th and 95th percentiles. Baseline drawdown is the maximum difference in drawdown (dmax) under the baseline relative to no coal resource development. Areas within mine pit exclusion zones are excluded from further analysis. Some totals do not add up due to rounding.
Data: Bioregional Assessment Programme (Dataset 3, Dataset 4, Dataset 5)
Table 21 Area (km2) of landscape classes in the ‘Groundwater-dependent ecosystem (GDE)’ landscape group potentially exposed to varying levels of drawdown due to additional coal resource development
The area potentially exposed to ≥0.2, ≥2 and ≥5 m additional drawdown is shown for the 5th, 50th and 95th percentiles. Additional drawdown is the maximum difference in drawdown (dmax) due to additional coal resource development relative to the baseline. Areas within mine pit exclusion zones are excluded from further analysis. Some totals do not add up due to rounding.
Data: Bioregional Assessment Programme (Dataset 3, Dataset 4, Dataset 5)
Table 22 Area (km2) of ‘Groundwater-dependent ecosystem (GDE)’ landscape classes in the Wyong River catchment potentially exposed to varying levels of drawdown due to additional coal resource development, based on regional parameter values
The area potentially exposed to ≥0.2, ≥2 and ≥5 m additional drawdown is shown for the 5th, 50th and 95th percentiles. Additional drawdown is the maximum difference in drawdown (dmax) due to additional coal resource development relative to the baseline. Areas within mine pit exclusion zones are excluded from further analysis. Some totals due not add up due to rounding.
Data: Bioregional Assessment Programme (Dataset 3, Dataset 4, Dataset 5)
Table 23 Area (km2) of ‘Groundwater-dependent ecosystem (GDE)’ landscape classes in the Wyong River catchment potentially exposed to varying levels of drawdown due to additional coal resource development, based on locally-constrained parameter values
The area potentially exposed to ≥0.2, ≥2 and ≥5 m additional drawdown is shown for the 5th, 50th and 95th percentiles. Additional drawdown is the maximum difference in drawdown (dmax) due to additional coal resource development relative to the baseline. Areas within mine pit exclusion zones are excluded from further analysis. Some totals do not add up due to rounding.
Data: Bioregional Assessment Programme (Dataset 3, Dataset 4, Dataset 5)
3.4.4.2.2 Surface water
Surface water hydrological response variables that represent change in the recurrence interval of overbench and overbank flows were used in the receptor impact model for ‘Forested wetland – riverine forest’. This model applies to riverine forests along unregulated rivers in the Hunter River basin, and is not considered appropriate for riverine forests along the regulated river. It is also not applicable to coastal swamp forested wetlands in the Macquarie-Tuggerah lakes basin, which comprise different tree species and could respond differently to changes in hydrology. There are 28.7 km2 of coastal ‘Forested wetland’ within the zone.
Overbench flows and overbank flows form part of the high flow end of the flow regime and enhance the hydrological connectivity between the floodplain and alluvial aquifers (Section 3.4.3.1). For the purposes of the ‘Forested wetland – riverine forest’ receptor impact model, overbench flows are assumed to occur on average about 3 times a year, which corresponds to a recurrence interval of 0.33 years; and overbank flows are flood flows and assumed to occur on average once every 3 years (recurrence interval of 3 years). At each surface water model node, the flow rates corresponding to these recurrence intervals define the thresholds for assessing the impact of additional coal resource development on the recurrence interval of overbench and overbank flows.
Most of the riverine forests potentially subject to changes in overbench or overbank flows due to additional coal resource development are located along the Goulburn River, Wollombi Brook and the Hunter Regulated River. Of the 29.1 km2 of ‘Forested wetland – riverine forest’ within the zone, changes in projected foliage cover cannot be quantified for 15.4 km2 because the changes in hydrology due to additional coal resource development were not able to be quantified in the reaches along which they occur.
For the reaches where surface water modelling results are available, 8.5 km2 of riverine forest are predicted to experience decreases in overbench and overbank flows. The predicted changes are generally small (i.e. fewer than 0.2 events per year decrease for overbench and fewer than 0.1 events per year decrease for overbank). A reduction of 0.2 means one fewer event every 5 years; a reduction of 0.1 is one fewer event every 10 years. Based on the median estimates from the modelling, it is unlikely that the frequency of overbench or overbank flows will change due to additional coal resource development (Figure 52, Figure 53, Figure 54, Figure 55) over the short or long term. The area of riverine forest potentially subject to a decrease in overbench flows of one event every 5 years, based on the median result, is 0.3 km2 in the 30-year period preceding 2042 (Table 24) and 0.2 km2 in the 30-year period preceding 2102 (Table 24). None of the riverine forest, for which changes can be quantified, is predicted to experience decreases in overbank flows of one event every 5 years due to additional coal resource development, but 0.2 km2 could experience a loss of one overbank flow event every 10 years (Table 25).
Overall, there is only a small chance in a few areas that riverine forests along unregulated rivers in the Hunter river basin will be impacted by changes in overbench or overbank flow due to additional coal resource development. The risk to riverine forests along the Hunter Regulated River cannot be determined with much certainty, although modelling results do show similar changes in the overbench (Figure 52 and Figure 53) and overbank (Figure 54 and Figure 55) hydrological response variables along the regulated reaches. The uncertainty associated with streamflow in a regulated river is reflected by greying out these reaches.
The Hunter Regulated River is greyed out because the receptor impact model was explicitly developed for riverine forests on unregulated rivers in the Hunter river basin.
Data: Bioregional Assessment Programme (Dataset 1, Dataset 2, Dataset 4, Dataset 5)
The Hunter Regulated River is greyed out because the receptor impact model was explicitly developed for riverine forests on unregulated rivers in the Hunter river basin.
Data: Bioregional Assessment Programme (Dataset 1, Dataset 2, Dataset 4, Dataset 5)
The Hunter Regulated River is greyed out because the receptor impact model was explicitly developed for riverine forests on unregulated rivers in the Hunter river basin.
Data: Bioregional Assessment Programme (Dataset 1, Dataset 2, Dataset 4, Dataset 5)
The Hunter Regulated River is greyed out because the receptor impact model was explicitly developed for riverine forests on unregulated rivers in the Hunter river basin.
Data: Bioregional Assessment Programme (Dataset 1, Dataset 2, Dataset 4, Dataset 5)
Table 24 Cumulative area (km2) of forested wetlands potentially exposed to changes in recurrence of overbench flows (EventsR0.3) due to additional coal resource development in the years 2042 and 2102
EventsR0.3 is the mean annual number of events with a peak daily flow exceeding the threshold (the peak daily flow in flood events with a return period of 0.3 years as defined from modelled baseline flow in the reference period (1983 to 2012)). This metric is designed to be approximately representative of the number of overbench flow events in future 30-year periods. This is typically reported as the maximum change due to additional coal resource development. Some totals do not add up due to rounding.
Data: Bioregional Assessment Programme (Dataset 5)
Table 25 Cumulative area (km2) of forested wetlands potentially exposed to changes in recurrence of overbank flows (EventsR3.0) due to additional coal resource development in the years 2042 and 2102
aAreas include coastal forested wetlands, but receptor impact model only applies to riverine forests along unregulated rivers in the Hunter river basin.
EventsR3.0 is the mean annual number of events with a peak daily flow exceeding the threshold (the peak daily flow in flood events with a return period of 3.0 years as defined from modelled baseline flow in the reference period (1983 to 2012)). This metric is designed to be approximately representative of the number of overbank flow events in future 30-year periods. This is typically reported as the maximum change due to additional coal resource development. Some totals do not add up due to rounding.
Data: Bioregional Assessment Programme (Dataset 5)
3.4.4.3 Potential ecosystem impacts
As described in Section 3.4.2, the ‘Spring’, ‘Semi-arid woodland’, ‘Heathland’ and ‘Grassy woodland’ landscape classes within the ‘GDE’ landscape group are very unlikely to be impacted. ‘Rainforest’ is also very unlikely to be impacted although there are knowledge gaps with respect to ‘Rainforest’ mapped over alluvium. Impacts on the ‘Freshwater wetland’ landscape class are a knowledge gap. In this section the potential ecosystem impacts on ‘Wet sclerophyll forest’, ‘Dry sclerophyll forest’ and ‘Forested wetland’ landscape classes are discussed.
3.4.4.3.1 Wet and dry sclerophyll forests
The receptor impact model for wet and dry sclerophyll forests identified relationships between the hydrological response variables described in Section 3.4.4.2 and projected foliage cover (m2/m2).
Median estimates of the projected foliage cover under the baseline and CRDP futures ranged from 0.25 to 0.35 (Figure 56). There was great uncertainty surrounding absolute values of projected foliage cover; there was a 90% probability that projected foliage cover in wet sclerophyll forests and dry sclerophyll forests in the zone could be in the range of less than 0.1 to more than 0.7 in both periods.
The median estimate from modelled changes in projected foliage cover in the 30-year periods preceding both 2042 and 2102 was that there would be no change compared to the baseline period (Figure 56). This is consistent with the small modelled changes in drawdown described in Section 3.4.4.2. Of the 18.8 km2 of wet sclerophyll forests and dry sclerophyll forests in the zone, it is predicted less than 2 km2 might experience an increase in projected foliage cover more than 0.03 (95th percentile difference) and that less than 5 km2 might experience a decrease in projected foliage cover more than 0.03 (5th percentile difference). A change in projected foliage cover of 0.03 represents 10% for the median estimate of projected foliage cover of 0.3. Hence, these changes are small in terms of foliage but would have flow-on effects to flower production, nectar production and nectar-feeding animals.
Overall, the modelled results suggest little detectable impact on the condition of wet sclerophyll forests and dry sclerophyll forests in the Hunter subregion due to additional coal resource development but that there is very high uncertainty surrounding estimates of absolute values. There is a 5% chance that 10 to 15 km2 of mainly dry sclerophyll forests in the Macquarie-Tuggerah lakes basin will be subjected to an adverse ecological impact, although the risk is much reduced when the groundwater modelling results are constrained by local hydrogeological information (see companion product 2.6.2 for the Hunter subregion (Herron et al., 2018); Section 3.3.2). Based on the qualitative model (see Section 2.7.4.3 in companion product 2.7 for the Hunter subregion (Hosack et al., 2018a)) decreases in projected foliage cover will result in declines in shade, habitat structure, nectar production, nectar consumers, predators, sap- and leaf-eating insects, gliders and koalas. The qualitative model also predicted that insects would increase as a consequence of a release from predation pressure.
Data: Bioregional Assessment Programme (Dataset 6)
3.4.4.3.2 Forested wetlands – riverine forests
The receptor impact model for ‘Forested wetland – riverine forest’ establishes relationships between the hydrological response variables described in Section 3.4.4.2 and projected foliage cover for riverine forests in the zone, excluding the coastal forests. Only about 16 km2 of riverine forests were able to be modelled because some areas of riverine forests are on stream reaches where hydrological changes at surface water model nodes were not able to be interpolated. Median estimates of the projected foliage cover under the baseline and CRDP futures range from 0.10 to 0.15 (Figure 57), but there is considerable variability around local factors that influence the condition of the canopy of riverine forested wetlands. Experts considered that projected foliage cover could vary between 0.05 to more than 0.60 in both periods, with marginally more projected foliage cover under the baseline than under the CRDP.
The median estimates from modelled changes in projected foliage cover in the 30-year periods preceding both 2042 and 2102 indicate virtually no change compared to the baseline period. This is consistent with the small modelled changes in drawdown, overbench flow and overbank flow described in Section 3.4.4.2. Of the 16 km2 of ‘Forested wetland’ in the zone that were modelled, less than 2 km2 are predicted to experience an increase in projected foliage cover of more than 0.02 (95th percentile difference) and less than 7 km2 are predicted to experience a decrease in projected foliage cover of more than 0.05 (5th percentile difference).
Overall, the modelled results suggest little impact on the condition of riverine forest in the Hunter subregion due to additional coal resource development. Areas of riverine forest associated with larger decreases in the frequency of overbank and overbench flows, such as along parts of the Goulburn River, are ‘more at risk of ecological and hydrological changes’. Based on the qualitative model (see Section 2.7.4.2 in companion product 2.7 for the Hunter subregion (Hosack et al., 2018a)) any decreases in projected foliage cover will reduce shade, habitat structure, bank stability, and orchids and fungi. The predicted decline in tree cover could benefit shrubs and herbaceous vegetation, with flow-on benefits to wombats, nectar production and nectar consumers. The magnitude of the impact and implications for the persistence of riverine forests in these more at risk areas will depend on local factors, influencing their current condition.
The receptor impact model was not developed for coastal ‘Forested wetland’ within the Macquarie-Tuggerah region, which comprise mainly the Keith (2004) vegetation class ‘Coastal Swamp Forests’ with some ‘Coastal Floodplain Wetlands’. The qualitative model, which applies to all ‘Forested wetland’ (see Section 2.7.4.2 in companion product 2.7 for the Hunter subregion (Hosack et al., 2018a)) indicates that where ‘Coastal Swamp Forests’ and ‘Coastal Floodplain Wetlands’ are subject to large drawdown or significant alterations to surface flow, similar changes could be expected as for riverine ‘Forested wetland’, including a decline in tree cover that might benefit shrubs and herbaceous vegetation, with flow-on benefits to nectar production and nectar consumers. Nectar-bearing flowers of the dominant trees are an important food source for flying-foxes, arboreal marsupials and birds (OEH, 2017). Groundwater modelling predicted that up to 17.6 km2 of ‘Coastal Swamp Forests’ might be subject to drawdown greater than 0.2 m and as much as 5.4 km2 might be subject to drawdown greater than 2 m (Table 21) due to additional coal resource development.
Figure 58 summarises the receptor impact modelling in terms of a relative risk map for ‘Forested wetland – riverine forests’. Forested wetlands along the Goulburn River are identified as ‘at some risk of ecological and hydrological changes’ from additional coal resource development, with those forested wetlands in the vicinity of the proposed Bylong mine predicted to be ‘more at risk of ecological and hydrological changes’ than upstream and downstream reaches. Forested wetlands along Wollombi Brook are also ‘at some risk of ecological and hydrological changes’. There are some ‘Forested wetland – riverine forests’ in the Central Hunter and Lower Hunter where hydrological changes were not quantified that are close to mines, which could also be at risk. The regulated Hunter River and coastal forested wetlands are greyed out to reflect that the receptor impact model was not intended to predict changes in projected foliage cover in these areas.
Data: Bioregional Assessment Programme (Dataset 6)
‘More at risk’ refers to ‘more at risk of ecological and hydrological changes’; ‘At some risk’ refers to ‘at some risk of ecological and hydrological changes’; ‘Minimal risk’ refers to ‘at minimal risk of ecological and hydrological changes’.
Data: Bioregional Assessment Programme (Dataset 9)
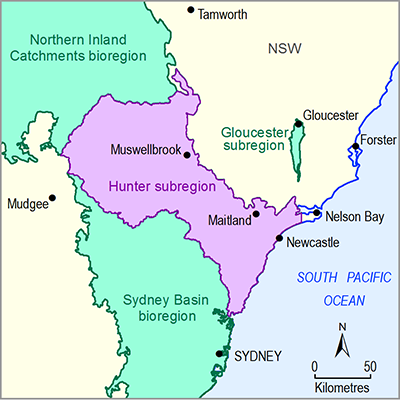
Product Finalisation date
- 3.1 Overview
- 3.2 Methods
- 3.3 Potential hydrological changes
- 3.4 Impacts on and risks to landscape classes
- 3.5 Impacts on and risks to water-dependent assets
- 3.6 Commentary for coal resource developments that are not modelled
- 3.7 Conclusion
- Citation
- Acknowledgements
- Contributors to the Technical Programme
- About this technical product