3.4.3.1 Description
The ‘Springs’ landscape group includes a number of spring types as detailed in Fensham et al. (2016). Broadly, Fensham et al. (2016) divided springs into two broad groups: discharge springs and recharge (also referred to as outcrop) springs (Figure 40).
Discharge springs occur where groundwater can discharge to the surface from a confined aquifer under hydrostatic pressure (i.e. the aquifer is artesian). Discharge through an aquitard may occur under a range of conditions including where:
- the confining aquitard is too thin or compromised in some other way (e.g. deep weathering lithological variations) to adequately confine the aquifer under prevailing hydrostatic pressures
- geological structures such as faults enable leakage towards the surface
- the aquifer abuts against basement rocks or another flow barrier, forcing groundwater flow upwards (Figure 40).
Discharge springs are located remote from the aquifer recharge zone, therefore, water flow to the spring is disconnected from local rainfall patterns and water chemistry tends to be alkaline with relatively high levels of dissolved solids (Fensham et al., 2016). Some discharge springs are characterised by groundwater scalds, where salts in the groundwater are precipitated from solution as water evaporates. In arid regions, these scalds are accentuated by the absence of periodic flushing due to overland flow, groundwater flow paths tend to be longer and springs are associated with regional-scale, confined groundwater systems (Figure 40).
Discharge springs are characterised by flat topography, mounded vents and absence of outcrop (Fensham et al., 2016). Discharge spring wetlands in the GAB are generally small, mostly less than 0.05 ha; however, a small number are greater than 1 ha (Fensham and Fairfax, 2003). Vegetation varies from site to site depending on moisture levels, but generally supports a ground layer of grasses, sedges and/or a mat of herbs. Discharge springs may host endemic flora and fauna, including ‘The community of native species dependent on natural discharge of groundwater from the Great Artesian Basin’, a nationally threatened ecological community, and various threatened species listed under the Commonwealth’s Environment Protection and Biodiversity Conservation Act 1999 (EPBC Act) and under Queensland’s Nature Conservation Act 1992 (Nature Conservation Act) (Fensham et al., 2010).
Discharge springs occur in areas where hydrostatic pressures in a confined aquifer are artesian, and where the overlying aquitard is compromised by stratigraphic thinning, geological structures, or the presence of a barrier that redirects regional groundwater flow. Recharge (or outcrop) springs are more commonly associated with unconfined aquifer conditions that occur at or near aquifer outcrop areas. Groundwater systems tend to be more local in scale.
Source: adapted from Queensland Department of Science, Information Technology and Innovation (Dataset 4), © The State of Queensland (Department of Science, Information Technology and Innovation) 2015.
Recharge (or outcrop) springs mainly occur in upland areas (Fensham et al., 2016) (Figure 40). Recharge springs form where groundwater discharges from the aquifer (commonly sandstone) in areas where the ground surface intersects with a saturated aquifer (Fensham et al., 2011; Fensham et al., 2016) in or near the outcrop of the aquifer. Water flow in recharge springs is dynamic and influenced by recent local rainfall patterns. Chemically, the water tends to be neutral or slightly acidic and contains low levels of dissolved solids (Fensham et al., 2016). Groundwater flow paths for recharge springs tend to be shorter and associated with more local-scale, unconfined groundwater systems (Figure 40).
Three distinct clusters of springs occur within the zone of potential hydrological change for the Galilee subregion:
- Doongmabulla Springs complex – including 187 individual vents that feed 160 separate wetlands. The springs form an isolated cluster of wetlands associated with the Carmichael River and its tributaries (Figure 41). Most wetlands (149 of 160) contain discharge springs and are located along the western edge of the complex. The western springs and spring groups are interpreted as being discharge springs based on flat topography, mounded vents and absence of outcrop (Fensham et al., 2016). Further east, recharge springs in the Doongmabulla Springs complex support 11 wetlands on gentle slopes of outcropping sandstone. The Doongmabulla Springs complex is described further in Section 3.4.3.1.1
- Permian springs cluster – springs where the source aquifer is a sedimentary unit of Permian age, principally the Colinlea Sandstone (part of the upper Permian coal measures), which has extensive outcrop on the footslopes of the Carnarvon Ranges. The Permian springs cluster includes both recharge (Albro group) and discharge springs (Lignum Spring, Storys Spring and Mellaluka Springs). Spring flows are low at Lignum Spring (about 0.5 L/min), moderate at Albro Springs (about 40 L/min) and high at Mellaluka Springs (about 1200 L/min). The Permian springs cluster does not support any spring endemic species (Fensham et al., 2016). Wetland vegetation is predominantly common and widespread, with no species of conservation significance. Fish fauna is limited to two common species
- Triassic springs cluster – recharge springs associated with sedimentary rocks of Triassic age, including gravity-fed outcrop springs emanating from fractures in local sandstone aquifers of the Dunda beds. The springs have all been heavily modified (Fensham et al., 2016), including two of the three Hector Springs that have been excavated to provide access for cattle watering. The plants and invertebrates that inhabit these springs are all common and widespread wetland species (Fensham et al., 2016). None are of conservation significance. The Triassic springs cluster does not include springs associated with the Doongmabulla Springs complex.
Figure 41 Doongmabulla Springs complex highlighting the name and location of individual spring vents
Data: Queensland Herbarium, Department of Science, Information Technology, Innovation and the Arts (Dataset 5); Queensland Department of Natural Resources and Mines (Dataset 6); Queensland Department of State Development, Infrastructure and Planning (Dataset 7)
3.4.3.1.1 Doongmabulla Springs complex
As outlined in companion product 2.3 for the Galilee subregion (Evans et al., 2018b), the identity of the source aquifer for the Doongmabulla Springs complex has been contentious and the subject of previous scientific dispute. Competing arguments for different source aquifer interpretations are presented by Currell (2016), Currell et al. (2017), Fensham et al. (2016), JBT Consulting (2015) and Webb (2015). Expert hydrogeological arguments (both for and against) provided as part of legal actions in the Queensland Land Court about the source aquifer for Doongmabulla Springs are summarised in Land Court of Queensland (2015a; 2015b). Importantly, in the context of this BA, assessing the potential impacts due to additional coal resource development on the Doongmabulla Springs complex depends on the interpretation of the source aquifer(s).
The groundwater source interpretation for the Doongmabulla Springs complex is based on various datasets, including sparse groundwater level and hydrochemistry data, regional surface and subsurface geological mapping, some drill-hole information and outputs from groundwater modelling. While there are noted points of contention (Land Court of Queensland 2015a; 2015b), the two interpretations for the source aquifer of the Doongmabulla Springs complex are summarised as:
- Upper Permian coal measures source aquifer – Webb (2015) proposed that a significant contribution to groundwater discharge at Doongmabulla Springs is provided by the upper Permian coal measures, principally the Colinlea Sandstone. In this interpretation, geological structures such as faults and fractures provide connectivity from the deep upper Permian coal measures (here over 500 m below surface) through the relatively low-permeability Rewan Group aquitard to the Clematis Group aquifer and other near-surface hydrogeological units. Supporting evidence for this interpretation includes potentiometric data for the Colinlea Sandstone, which indicate convergence of groundwater flow in this unit towards the springs location, evidence of faults in the area around the proposed Carmichael Coal Mine from seismic reflection data, as well as scant evidence of major confining layers within the Triassic sandstones of this area (Currell et al., 2017). Further, some analysis of existing groundwater chemistry data had proven inconclusive in identifying the source aquifer for some of the vents in the Doongmabulla Springs complex (Webb, 2015).
- Clematis Group source aquifer – JBT Consulting (2015) and GHD (2013a) considered the main spring source aquifer to be the Clematis Group (Clematis Sandstone and Warang Sandstone), which lies stratigraphically above the Rewan Group aquitard. This interpretation was based on available geological data and potentiometric mapping for the Clematis Group and other hydrogeological units in the area, as well as the known geomechanical and hydrogeological properties of the Rewan Group, and the low potential for faults to cross-cut the entire thickness of the Rewan Group aquitard. Recharge was conceptualised to occur from outcrop in nearby ranges (specifically Darkies Range), mainly to the north of the springs complex.
From analysis of the available hydrogeological data and information, the BA for the Galilee subregion (see companion product 2.3 for the Galilee subregion (Evans et al., 2018b)) considered the more likely source aquifer for the Doongmabulla Springs complex to be primarily the Clematis Group aquifer, with potentially some contribution of flow from the Dunda beds aquifer. The main reasons supporting this interpretation are:
- Potentiometric contour mapping for the Clematis Group aquifer indicates that regional groundwater flow focuses towards Dyllingo Creek and the Carmichael River valley, with the Doongmabulla Springs complex (companion product 2.1-2.2 (Evans et al., 2018a) and companion product 2.3 (Evans et al., 2018b) for the Galilee subregion) occurring at the lowest points in the potentiometric mapping for Clematis Group aquifer.
- Potentiometric contour mapping for upper Permian coal measures (specifically the Colinlea Sandstone) suggests that groundwater flow also focuses towards Carmichael River valley as well as parts of the Belyando River valley, east of Doongmabulla Springs complex (companion product 2.1-2.2 (Evans et al., 2018a) and companion product 2.3 (Evans et al., 2018b) for the Galilee subregion). However, in the vicinity of the Doongmabulla Springs complex the upper Permian coal measures are vertically separated from shallower aquifers such as the Clematis Group by the significant thickness (several hundred metres) of the Rewan Group aquitard. In contrast, the upper Permian coal measures are known to occur beneath Cenozoic sediment cover in the Belyando River valley in the vicinity of the proposed Carmichael Coal Mine. The Mellaluka Springs complex occurs in this area of the floodplain and represents a surface expression of discharge from these deeper Permian units.
- While there is hydraulic potential for some upwards leakage to occur across the Rewan Group aquitard from the upper Permian coal measures (for discussion see Section 2.3.2 in companion product 2.3 for the Galilee subregion (Evans et al., 2018b)), the Clematis Group aquifer has a more direct groundwater flow path and proximal hydrological connection to the Doongmabulla springs complex. Also, the bulk horizontal hydraulic conductivity of the Clematis Group aquifer is much higher, relative to the vertical conductivity of the underlying aquitard. Thus, the relatively high conductivity of the Clematis Group aquifer will likely permit a much greater volume of groundwater to be transmitted towards the springs than what may occur through the underlying aquitard.
- Several of the eastern-most spring groups included as part of the Doongmabulla Springs complex occur near areas of the Dunda beds outcrop (Figure 41). These are areas where the Clematis Group aquifer does not occur; hence, it is likely that the source aquifer for these springs is the Dunda beds aquifer (and not the Clematis Group aquifer).
Although the Assessment team considers that the available evidence supports the Clematis Group aquifer as the most likely groundwater source for the Doongmabulla Springs complex, there are some discrepancies with the available data in and around the zone of potential hydrological change (e.g. variability in the mapped extents of various geological units of the Galilee Basin (see Section 3.3)). In addition, the analysis undertaken for this BA has highlighted key geoscientific data and knowledge gaps, which are discussed further in Section 3.7, as well as in companion product 2.1-2.2 (Evans et al., 2018a) and companion product 2.3 (Evans et al., 2018b) for the Galilee subregion. Future targeted research to address these gaps would greatly assist with future management of the springs complex and better understanding its response to predicted levels of groundwater drawdown due to additional coal resource development. As an example, Section 3.5 highlights how remote sensing data have been utilised to determine the distribution and permanence of near-surface water at the Doongmabulla Springs complex over the past 30 years.
The BA conceptualisation of the Doongmabulla Springs complex, as detailed in this product and companion product 2.3 for the Galilee subregion (Evans et al., 2018b), differs somewhat from the conceptualisations published previously (as noted above). A key difference is that the BA conceptualisation emphasises how the characteristics of the aquifer source (e.g. confined or unconfined, and local versus regional flow system) may vary for different spring groups at different locations within the broader complex. For the spring complex as a whole, there is potential for contributions from local groundwater systems from unconfined portions of the Clematis Group and Dunda beds aquifers, as well as contributions from the regional groundwater flow system that occurs where the Clematis Group aquifer is confined by the Moolayember Formation aquitard.
The conceptual hydrogeological analysis undertaken for this BA indicates that the discharge springs (mound springs) in the western part of the Doongmabulla Springs complex (Figure 41) are fed by groundwater discharge from the confined Clematis Group aquifer by leakage through the overlying Moolayember Formation aquitard and thin alluvial cover. This occurs where the integrity of the Moolayember aquitard is compromised by decreased thickness of the aquitard towards its eastern contact with the Clematis Group, the variable effects of weathering and any local influence of geological structures. In the confined parts of the aquifer regional groundwater flow from westerly and southerly directions is focused towards the discharge springs. Outcrop springs in the Doongmabulla Springs complex occur on or near areas of outcrop for the Clematis Group and Dunda beds (see Figure 41). Local-scale groundwater systems may occur in these outcrop areas, with recharge occurring in nearby hills immediately east and north of the springs. Flow towards the outcrop springs is predominantly from the south and north, focused towards the valley of the Carmichael River. For the easternmost outcrop springs (e.g. Surprise and Dusk springs, Figure 41) the Dunda beds aquifer is interpreted as the most likely source aquifer. Here, it outcrops in nearby hills and underlies the alluvium in this part of the Carmichael River valley.
Discharge from all springs at the surface contributes directly to baseflow in the Carmichael River and to permanent pools (Section 3.5) in nearby drainage channels. There is also potential for groundwater from the Clematis Group and Dunda beds aquifers to discharge directly into the surficial alluvium in the Carmichael River valley (Figure 41).
3.4.3.2 Potential hydrological impacts
Springs are not represented directly in the AEM developed for the BA for the Galilee subregion (companion product 2.6.2 for the Galilee subregion (Peeters et al., 2018)). Instead, drawdown is estimated by comparing model layer drawdown at the location of the spring complex. This is a conservative approach that is considered to be appropriate for a regional-scale assessment as it may over estimate groundwater drawdown, given that groundwater discharge via the springs is not specifically included in the model.
The impact and risk analysis assumes that potential impacts and risks to springs in the assessment extent are associated with additional drawdown in the source aquifers represented by the following AEM layers:
- Doongmabulla Springs complex – as outlined above, drawdown in the Clematis Group aquifer model layer (using both original and alternative conceptualisations implemented in the model, as discussed in Section 3.3.2.1.1)
- Permian springs cluster – drawdown in the upper Permian coal measures. The Permian springs cluster is listed in the ‘Galilee-Permian’ region in the DSITIA spring location dataset (Queensland Herbarium, Department of Science, Information Technology, Innovation and the Arts, Dataset 5)
- Triassic springs cluster – drawdown in the Dunda beds source aquifer was not specifically estimated with the AEM. However, drawdown in the Dunda beds is likely to fall within the overall range predicted for the upper Permian coal measures and Clematis Group model layers, as the Dunda beds overlie the Rewan Group aquitard and underlie the Clematis Group aquifer. The Assessment team did not consider it valid to present drawdown results for these springs as the source aquifer was not explicitly modelled
- Other GAB springs – GAB springs nearest to the zone of potential hydrological change with source aquifers in the Eromanga Basin comprise part of the Barcaldine Springs supergroup. These springs occur outside of the modelled impact (Figure 42a) for the alluvial layer (which can overlie the GAB aquifer outcrop) and the Clematis Group aquifer (which underlies and is separated from GAB aquifers by the Moolayember Formation aquitard).
Additional drawdown with at least a 5% chance of exceeding 0.2 m is predicted in the source aquifer of 188 springs within the zone (out of the 1559 springs in the Galilee assessment extent) (Figure 42 and Figure 43). Modelled drawdown estimates for the main spring complexes within the zone are discussed further below.
3.4.3.2.1 Doongmabulla Springs complex
Using the modelled drawdowns for the Clematis Group aquifer from the original AEM conceptualisation, a total of 181 of the 187 springs of the Doongmabulla Springs complex have at least a 5% chance of exceeding 0.2 m of drawdown (Figure 43 and Table 20). As detailed in Section 3.3.2.1.1, the original AEM conceptualisation allows for drawdown from the mines to propagate to the Clematis Group aquifer via two distinct pathways, one through dewatering the deeper upper Permian coal measures and the other via draining the upper Quaternary alluvium and Cenozoic sediment aquifer. The median additional drawdown in excess of 0.2 m under this conceptualisation is also predicted to affect 181 springs in this complex. There is a 5% chance that 120 springs in this complex will experience drawdown in excess of 2 m in the Clematis Group aquifer, although there are no springs in the Doongmabulla Springs complex that are predicted to experience additional drawdown in excess of 5 m.
Using an alternative AEM conceptualisation, which allows for drawdown to propagate to the Clematis Group aquifer only via the deeper pathway from the upper Permian coal measures (see Section 3.3.2.1.1 for details), the groundwater modelling also predicts that 181 springs in the Doongmabulla Springs complex have a 5% chance of additional drawdown in excess of 0.2 m (Figure 43 and Table 20). However, estimates using this alternative AEM conceptualisation indicate that there are no springs in this complex predicted to experience median additional drawdown in excess of 0.2 m.
As outlined in Section 3.3.2.1.1, it is likely that the results from the original AEM conceptualisation over estimate drawdown in areas where the actual areal distribution and thickness of the alluvial layer is more restricted than implemented in the AEM, such as near the Doongmabulla Springs complex. This recognition led to the development of the alternative conceptualisation, which only allows for drawdown to occur from the deeper upper Permian coal measures through the regional aquitard pathway (i.e. no drawdown propagation occurs from the mines via the alluvium). The Assessment team considers that the alternative conceptualisation provides a better estimate of drawdown in the Clematis Group in areas where the alluvial layer does not occur as an extensive sheet-like layer that is in direct (and unimpeded) contact with the mining areas. However, this hypothesis would need to be further tested through a combination of field work and modelling, including analysis of groundwater samples for environmental tracers and isotopes, geophysical surveying, and more detailed local-scale groundwater modelling that incorporates any new understandings and conceptualisations derived from field work (see Section 3.7 for further discussion and recommendations).
3.4.3.2.2 Permian springs cluster
The median modelling result indicates that drawdown at all seven of the springs that access the upper Permian coal measures in the zone will exceed 0.2 m (Figure 43 and Table 20). The range of model predictions indicates that the number of springs predicted to experience additional drawdown in excess of 0.2, 2 and 5 m is between five and seven springs. Additional drawdown is very likely to exceed 5 m at five springs in the Permian springs cluster (Lignum, Storys and Mellaluka springs).
3.4.3.2.3 Triassic springs cluster
Drawdown for the 12 Triassic springs in the zone cannot be reliably estimated by the AEM (as the source aquifer is not specifically modelled), but is likely to fall within the range predicted for the Clematis Group model layer (Figure 43 and Table 20). Fensham et al. (2016) reported that the Greentree Spring has been inactive (dry) for a considerable time, possibly since the turn of the 20th century. In contrast, Hunter Springs is only known to have dried up once in living memory, at the end of a major drought in the 1940s (Fensham et al., 2016).
3.4.3.2.4 Other Great Artesian Basin (GAB) springs
None of the 1353 GAB springs with source aquifers that are part of the Eromanga Basin occur in the zone of potential hydrological change (Figure 42 and Table 20). These GAB aquifers are part of the hydrostratigraphic sequence of the Eromanga Basin (which is geologically younger and overlies much of the Galilee Basin in the subregion). Fensham et al. (2016) noted that 15% of GAB discharge springs included in the Barcaldine Springs supergroup are currently inactive.
As outlined in companion product 2.6.2 for the Galilee subregion (Peeters et al., 2018), caution should be used when comparing results from different groundwater models for a number of reasons including differences in model assumptions, conceptualisations, operation of modelling code and construction. Full conceptualisation details of other groundwater models in the Galilee subregion are described in companion product 2.6.2 for the Galilee subregion (Peeters et al., 2018) and summarised here for some selected springs.
Numerical groundwater modelling associated with the proposed Carmichael Coal Mine and Rail Project has identified potential impacts to the Doongmabulla Springs and Mellaluka Springs complexes (GHD, 2013b). Previous estimates of pressure reductions at various springs during the operational and post-closure phases of the Carmichael Coal Mine are up to 0.19 m at Joshua Spring during the operational phase, 0.12 m at Moses Springs and 0.05 m at Little Moses Spring (GHD, 2013b). These estimates are less than estimates of additional drawdown made with the original AEM conceptualisation used in the regional groundwater modelling approach for the BA, and are consistent with estimates made using the alternative conceptualisation (Figure 43 and Table 20). However, these results did not account for the potential hydrological effects of all of the proposed coal mines included in the additional coal resource development for this BA.
Previously predicted pressure reductions in the Mellaluka Springs complex during mine operations are 1.14 m at Mellaluka Springs, 2.34 m at Storys Spring and 8.22 m at Lignum Spring (Table 5 in GHD, 2013b). The pressure reduction becomes greater during the post-closure phase (likely to be around 2070) with pressure reductions of 9.07, 13.4 and 25.6 m, respectively, at the three springs (GHD, 2013b, Table 5). These estimates are less than the median additional drawdown predicted at Mellaluka Springs of approximately 60 m (e.g. model receptor GAL_043, confined upper Permian source aquifer) by either the original or alternative groundwater model conceptualisation reported in this Assessment (Figure 28 in companion product 2.6.2 for the Galilee subregion (Peeters et al., 2018)).
All results shown are based on the original conceptualisation used to assess drawdown using the analytic element model (AEM) for the Galilee subregion.
GAB = Great Artesian Basin
Data: Queensland Herbarium, Department of Science, Information Technology, Innovation and the Arts (Dataset 5); Bioregional Assessment Programme (Dataset 8, Dataset 9)
This analysis assumes that additional drawdown at springs in the Doongmabulla Springs complex is due to drawdown in the Clematis Group aquifer model layer, and drawdown at springs in the Permian springs cluster is due to drawdown in the upper Permian coal measures model layer. The modelling results for the Doongmabulla Springs complex are shown for both the original AEM conceptualisation (a) and the alternative AEM conceptualisation (b), as explained in the accompanying text.
Data: Queensland Herbarium, Department of Science, Information Technology, Innovation and the Arts (Dataset 5); Bioregional Assessment Programme (Dataset 8)
Table 20 ‘Springs’ landscape group: number of springs (number) potentially exposed to varying levels of additional drawdown in the zone of potential hydrological change
This analysis assumes that additional drawdown at springs in the Doongmabulla Springs complex is due to drawdown in the Clematis Group aquifer model layer, and drawdown at springs in the Permian springs cluster is due to drawdown in the Betts Creek beds model layer. Drawdown at springs in the Triassic springs cluster cannot be estimated by the analytic element model (AEM) as the source aquifer was not specifically included in the model design.
The original conceptualisation for the AEM allows for two drawdown pathways (Section 3.3.2.1.1). These two pathways account for drawdown through the regional aquitard (Rewan Group) as well as near-surface drawdown/drainage through the surficial aquifer. As explained in this section and Section 3.3.2.1.1, the AEM results may be overly conservative for the Doongmabulla Springs complex. The alternative conceptualisation for the AEM only accounts for drawdown through the regional aquitard (Section 3.3.2.1.1). Further work would be required (as outlined in Section 3.7) to confirm if results from a regional-scale model can adequately account for local-scale influences on groundwater flow at the Doongmabulla Springs complex.
GAB = Great Artesian Basin; NA = not available
Data: Queensland Herbarium, Department of Science, Information Technology, Innovation and the Arts (Dataset 5); Bioregional Assessment Programme (Dataset 8)
3.4.3.3 Potential ecosystem impacts
A qualitative mathematical model was developed that described the general dynamics of the aquatic community associated with springs in the zone of potential hydrological change (see Section 2.7.3 in companion product 2.7 for the Galilee subregion (Ickowicz et al., 2018)). The experts identified that a critical factor in preserving the aquatic community is the rate of groundwater flow that maintains a damp or submerged state in the area around the spring vent and any associated spring pools down-gradient, such that this surface area does not become dry. An increase in water depth above this threshold[1] supports a wetted-area regime around the perimeter and downstream of the spring that is beneficial to emergent vegetation, the building of peat mounds, tail vegetation (i.e. vegetation at the outfall or tail end of a spring) and groundwater-dependent vegetation. Within the free-water area of a spring, an increase in water depth enhances primary production (i.e. phytoplankton, macrophytes and benthic algae) and habitat for aquatic grazers.
The modelled estimates of expected pressure reduction in aquifers within the ‘Springs’ landscape group indicate a high potential for ecosystem impact. The impact varies probabilistically with location but is predicted for the Doongmabulla Springs complex, Permian springs cluster and Triassic springs cluster. However, as explained in detail in companion product 2.7 for the Galilee subregion (Ickowicz et al., 2018), it was not possible to develop receptor impact models for the ‘Springs’ landscape group in the Galilee subregion.
At the Doongmabulla Springs complex, the predicted level of pressure reduction will affect water flows and decrease water availability to GDEs. However, the long-term impact of this pressure reduction on the springs and spring wetlands and the constituent biota is unclear or contestable. Section 3.5.2.6 provides further discussion on water-dependent assets at the Doongmabulla Springs complex and potential ecosystem impacts may affect these assets.
For the Permian springs cluster, including Mellaluka Springs, the predicted pressure reductions during mine operations are likely to reduce flows at the surface for all springs within the cluster. Hydrological impacts potentially will result in the loss of ecological functioning of these springs, and also affect their use as a reliable pastoral water supply.
At the Triassic springs cluster, drawdown cannot be reliably estimated by the AEM, but is likely to fall within the range predicted for the Clematis Group model layer. Therefore, the ecological consequences of predicted pressure reductions are less certain at the Triassic springs cluster.
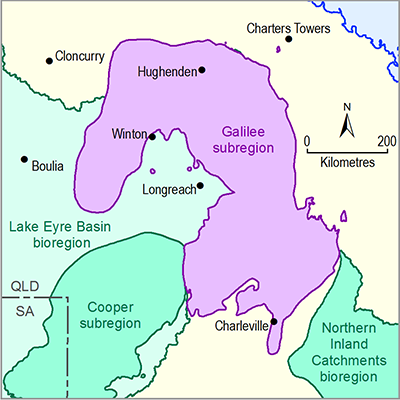
Product Finalisation date
- 3.1 Overview
- 3.2 Methods
- 3.3 Potential hydrological changes
- 3.4 Impacts on and risks to landscape classes
- 3.4.1 Overview
- 3.4.2 Landscape classes that are unlikely to be impacted
- 3.4.3 'Springs' landscape group
- 3.4.4 'Streams, GDE' landscape group
- 3.4.5 'Streams, non-GDE' landscape group
- 3.4.6 'Floodplain, terrestrial GDE' landscape group
- 3.4.7 'Non-floodplain, terrestrial GDE' landscape group
- References
- Datasets
- 3.5 Impacts on and risks to water-dependent assets
- 3.6 Commentary for coal resource developments that are not modelled
- 3.7 Conclusion
- Citation
- Acknowledgements
- Contributors to the Technical Programme
- About this technical product