The coal mine and CSG operations, and the critical water pathways, are contained entirely within the Gloucester PAE (McVicar et al., 2014, p. 17, Section 1.1.2.1; Parsons Brinckerhoff, 2012, p. 30; SRK, 2010, p. 45).
2.3.2.2.1 Geology
The basin is an elongated north-trending sedimentary basin, about 55 km long and up to 15 km wide, containing up to 2500 m of faulted, deformed and eroded coal-bearing Permian sedimentary and volcanic rocks (McVicar et al., 2014, p. 43, Section 1.1.3). The strata in the basin are relatively flat in the central portion, and are steeply dipping and faulted toward the flanks (Figure 6).
The near-surface layers are the Leloma Formation and Crowthers Road Conglomerate within the Craven Subgroup. The upper parts consist of interbedded sandstones, siltstones, claystones and conglomerates that form poor-yielding confined aquifers. However, where they are fractured (from about 100 m and below) they are categorised as water-bearing formations. The other members of the subgroup contain sandstones and conglomerates interbedded with coal seams. Due to faulting and erosion these other members and their coal seams may outcrop where the strata dip steeply enough.
Source: Roberts et al. (1991). This figure is not covered by a Creative Commons Attribution licence. It has been reproduced with the permission of NSW Trade & Investment.
The next layers are the Speldon Formation and Avon Subgroup, formed by marine transgressions and coastal plain environments, containing sandstone and siltstone layers with interbedded coal seams. These layers contain the most saline groundwater in the basin, due to their marine origin (see Parsons Brinckerhoff, 2012, p. 96, Table 8-3; p. 100, Table 8-5), about double the salinity of groundwater contained in the SRL. The Dewrang Group has similar origins to the Avon Subgroup but contains coarse-grained sandstones and conglomerates, and contains the Duralie Road and Weismantels formations worked at Duralie Coal Mine. The base unit of Alum Mountain Volcanics contains volcanic and sedimentary rocks, and is considered impermeable to water.
During the review of the geological structure of the Gloucester Basin, it was determined that the existing structural framework dating back to the 1990s was inadequate and led to substantial uncertainty about the location and orientation of faults (see companion product 1.1 for the Gloucester subregion (McVicar et al., 2014)). As part of the work in this subregion, a first-order regional geological model was developed that was based on all available deep borehole lithologies and geophysical data. Major faults were included to account for the basin-scale architecture, while a probabilistic fault population model for sub-seismic faults was developed based on published data from other basins of similar origin. The details of the geological model are found in companion product 2.1-2.2 for the Gloucester subregion (Frery et al., 2018), and the fault population models are used in numerical modelling in companion product 2.6.2 for the Gloucester subregion (Peeters et al., 2018).
2.3.2.2.2 Hydrogeology
The hydrogeology with respect to water movement was divided into three units: alluvial aquifer, SRL, and alternating units of interburden and coal seams (see companion product 1.1 for the Gloucester subregion (McVicar et al., 2014, Section 1.1.4)).
Within the 7 to 15 m thick alluvial aquifer are the streams and rivers that pass through the subregion. The aquifer consists of gravel, sand, and clay layers and lenses, with a high range of conductivity locally (0.3 to 500 m/day), and is overall the most hydraulically conductive hydrogeological unit in the subregion (see companion product 1.1 for the Gloucester subregion (McVicar et al., 2014, Section 1.1.4 and Section 1.1.6)). The local water levels are shallow and within the first few metres of the land surface adjacent to the watercourses. These water levels are responsive to rainfall events and floods in the surface water catchments. Rivers and streams in the Gloucester subregion are mostly gaining, although the total water volume may be small relative to total streamflow (see companion product 1.1 for the Gloucester subregion (McVicar et al., 2014, Section 1.1.5 and Section 1.1.6)).
The SRL is locally confined and of lower hydraulic conductivity than the alluvial aquifer. The conductivity can be modelled as decreasing exponentially with depth (Parsons Brinckerhoff, 2013b, Figure 4-9). The water levels in the SRL have only limited response to rainfall seasonally, and there is only occasional response to individual rainfall events (see Parsons Brinckerhoff 2013a, p. 10–22, Figure A-6 to Figure A-30), although in some areas there may be greater connectivity and groundwater response (SKM, 2012). These conclusions indicate that recharge naturally occurs in the SRL and potentially to deeper layers. The hydraulic conductivity of the entire weathered zone is heterogeneous, with higher and lower conductivity domains associated with fault and fracture zones. The known aquifer zones occur to a maximum depth of 150 m but are mostly present in the upper 100 m.
The deeper interburden and coal seam layers are referred to as ‘water bearing’ rather than ‘aquifers’, which implies they are strata that yields a low amount of water. The reduction in hydraulic conductivity of both layer types is considered as exponentially decaying (SRK, 2010; Australasian Groundwater and Environmental, 2013; Heritage Computing, 2009), although the conductivity of the interburden layer is one or two orders of magnitude less than for a coal seam layer at the same depth.
As a closed basin, any rainfall recharge that occurs must result in storage within the geological basin or discharge to the surface via evaporation or to the stream via the alluvial aquifer. Recharge occurs to the alluvial aquifer and outcropping of shallow strata within the valley floor, and to deeper water-bearing zones around the flanks of the geological Gloucester Basin. Fluxes between layers are generally low with the greatest being from the SRL to alluvial aquifer in the valley floor. However, there is no consistent observed upward trend between layers in nested bores (see companion product 1.1 for the Gloucester subregion (McVicar et al. 2014, Section 1.1.4)).
Faulting and fracturing potentially play a major role in the groundwater flow paths from deeper to shallower layers. However, the role of faults and fracture zones is not consistent or well understood in the subregion as they have been reported to be both impediments to flow (URS, 2007; Parsons Brinckerhoff, 2012), and to have enhanced hydraulic conductivity (Parsons Brinckerhoff, 2013b; SRK, 2010) with chemical signatures indicative of upward flow of deep groundwater under natural conditions. Section 1.1.3 of companion product 1.1 of the Gloucester subregion (McVicar et al., 2014) states:
The present definition of the structural framework for the Gloucester Basin relies on: (i) sparse two-dimensional seismic reflection data, (ii) interpolation of surface geological mapping, (iii) correlation of coal seams from borehole data, (iv) observations in open-cut mines, and (v) geophysical surveys. The low density and poor resolution of the seismic data, the limited number of outcropping structures and the high degree of lateral stratigraphic variation in the basin result in significant uncertainty about the location and orientation of subsurface structural features.
Probabilistic modelling of inferred fractures shows that on a regional scale there is limited propagation of drawdown at the regional scale (companion product 2.6.2 of the Gloucester subregion (Peeters et al., 2018)); however, no actual well locations were specified at the time of this work and any local effects could not be addressed.
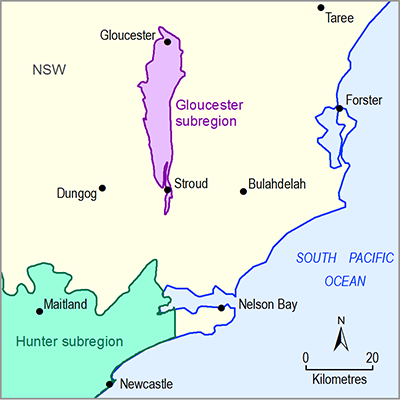
Product Finalisation date
- 2.3.1 Methods
- 2.3.2 Summary of key system components, processes and interactions
- 2.3.3 Ecosystems
- 2.3.4 Baseline and coal resource development pathway
- 2.3.5 Conceptual modelling of causal pathways
- Citation
- Currency of scientific results
- Acknowledgements
- Contributors to the Technical Programme
- About this technical product