- Home
- Assessments
- Bioregional Assessment Program
- Methods
- Impacts and risks
- 2 Design choices for the impact and risk analysis
2.1 Objectives
The BA methodology (Barrett et al., 2013) defines a bioregional assessment (BA) as a regional cumulative analysis that assesses potential impacts of coal resource development on water resources and water-dependent assets by comparing results for two possible futures. The baseline future includes all coal resource developments that commenced commercial production prior to December 2012. The coal resource development pathway (CRDP) future includes not only the baseline coal resource developments but also the additional coal resource development, new developments, or expansions of baseline operations that are expected to commence production after 2012. The primary focus of a BA is on the potential impacts on water resources and water-dependent assets that are attributable to this additional coal resource development.
In the Bioregional Assessment Programme (the Programme), the term ‘coal resource development’ specifically includes coal mining (both open-cut and underground) as well as coal seam gas (CSG) extraction. However, other forms of coal-related development activity, such as underground coal gasification and in situ microbial enhancement of coal-hosted gas resources, are not within the scope of the assessment.
A risk analysis requires the consideration of consequences of impacts as well as the likelihood of them occurring. In BAs, the consequences are reported for water resources and water-dependent assets that have been identified by the local communities and natural resource management agencies as having ecological, economic or sociocultural value. The overarching objective of the impact and risk analysis is to determine how the specified coal resource development may impact these water resources and water-dependent assets over both space and time given the existing understanding and uncertainty in the biophysical systems and coal resource development operations. BAs present the predicted biophysical consequences of coal resource development in terms of changes to hydrology and ecological variables. BAs do not assess the ecological or socioeconomic significance of these changes because this requires value judgments and non-scientific information that is outside the scope of BA.
At the heart of the impact and risk analysis is a conceptual chain of causation, where activities in the coal resource development within the biophysical context of the bioregion or subregion lead to hydrological changes (represented by hydrological response variables, such as groundwater drawdown), which in turn results in changes in the environment and ecology (represented by receptor impact variables, such as vegetation condition). Figure 5 illustrates this chain of causation and the conditional relationship between variables considered in the impact and risk analysis. The fundamental comparison between the two futures enables prediction of hydrological and ecological changes that are attributed to the additional coal resource development (D in Figure 5).
2.2 Design constraints
Three classes of constraints influenced the design of this methodology. Those imposed by:
- the BA methodology
- the complexity of the bioregions and assets
- good practice in risk assessment.
2.2.1 Constraints imposed by the bioregional assessment methodology
The objectives of the BA methodology (Barrett et al., 2013) are very challenging, and to date have not been achieved elsewhere. Among the specific constraints, the BA methodology:
- specifies that the analysis needs to assess direct, indirect and cumulative impacts, which is still a developing area in risk analysis
- requires assessing water-related impacts of coal resource development on assets. Impacts vary in space and time, so achieving this requires the ability to predict impacts spatially and temporally
- focuses on impacts related to water quantity and availability. Potential water quality impacts are limited to salinity, with other water quality impacts beyond the scope
- is explicit about assessing impacts for two futures: baseline and CRDP, which includes likely additional coal resource development.
- does not consider the value of assets beyond accepting that the community values the assets that they identified. The methodology predicts consequences (i.e. biophysical impacts such as hydrological or ecological changes), but does not address the significance of the predicted consequences.
2.2.2 Constraints imposed by complexity of the bioregions
The bioregions or subregions are often large and cover broad geographic extents, and the human and ecological systems are complex and diverse. Specific constraints include:
- The landscape is heterogeneous and may depend on various subsurface features. It is necessary to consider individual elements of a landscape within the broader system context and interactions to simplify and ensure the analysis is tractable.
- The scale, intensity and spatial extent of impacts will vary through time but high-resolution ecological and hydrological predictions are not currently feasible. Results at specific locations can be predicted for only a few time points.
- A large number of water-dependent assets were identified, and it is not possible to manually assess each asset individually within time frames that are useful for decision makers.
- Some of the hydrological changes that may occur after development may not have been observed before in an area, and there is therefore no empirical data to base inferences on.
- The complexity of the bioregion or subregion needs to be addressed within the operational constraints of the Programme. If a region can be ruled out from impact, halting further analysis ensures that resources can be concentrated on the most important areas.
- Ecological systems are complex and demonstration of causation is challenging so the unambiguous identification of direct, indirect and cumulative impacts is difficult.
2.2.3 Constraints imposed to achieve good practice in risk assessment
Good practice in risk assessments (Burgman, 2005; Suter, 2006) needs to be achieved so that the results are of high scientific quality, and are useful for managing risks. Specific constraints include that the impact and risk analysis needs to:
- be repeatable and falsifiable, and make predictions that can be validated over time through observation
- be complete in its coverage of the breadth of potential impacts on and risks to water resources and water-dependent assets within the scope of BA
- effectively integrate across available models and information, but also allow updates if models and information change or improve
- reliably represent and communicate the uncertainties embedded in the impact and risk analysis, and equally importantly identify where confidence in results is high.
2.3 Design choices
The design constraints described in the previous section define requirements for the impact and risk methodology: it must meet the objectives of the BA methodology while also being practical and respecting good practice. Making design choices that meet these requirements ensures that BAs are credible and timely and thus can constructively inform public debate and decision making.
The major design choices are:
- a dedicated hazard analysis
- a quantitative analysis of impacts and risks
- assessment of regional-scale cumulative impacts
- a focus on predictive uncertainty
- hydrological predictions for any location in the landscape
- decomposition of the predictions into conditionally independent components
- a landscape classification
- two assessment time points
- use of expert opinion where empirical data are limited
- qualitative mathematical modelling to estimate direct and indirect impacts and choose key variables
- automation of the analysis.
2.3.1 A dedicated hazard analysis
A hazard can be defined as a situation that in particular circumstances could lead to harm (The Royal Society, 1983) or alternatively considered as a substance’s or activity’s propensity for risk. Hazards are sometimes perceived to be solely a function of a substance’s intrinsic properties but, as emphasised in the definition above, they are more usefully conceptualised as a function of both the intrinsic properties of a substance and circumstance.
For example, co-produced water from CSG production may not ordinarily be considered hazardous, but if it is used carelessly to irrigate land it may cause detrimental changes to the soil chemistry and negatively impact agricultural production. Thus a substance’s intrinsically hazardous properties can often only be realised under a very specific set of circumstances. A hazard analysis should properly acknowledge both the intrinsic properties and the circumstances required in order for harm to be realised. The measure of the likelihood of these circumstances and the magnitude of the subsequent harm is a measure of risk. Put another way, a hazard becomes a risk only when there is a non-negligible probability of a manifestation of the hazard (Beer and Ziolkowski, 1995).
Hazard analysis is a structured process designed to identify the substances and circumstances surrounding an activity that cause harm; such an analysis provides a mechanism to rank potential hazards against a variety of criteria, but most usually the likelihood and severity of this harm. In this manner a hazard analysis prioritises tasks and resources within an assessment and provides the logical basis to support the breadth and detail of the analysis presented in the products. For example, a hazard that has low likelihood of occurring and little consequence may be reported as such (in the interests of transparency), but accorded fewer resources for the analysis compared to hazards with higher consequence and likelihood.
A carefully structured hazard analysis is a key component of a BA and needs to be completed to enable this essential ruling out, given the wide scope of potential risk endpoints. Significant commonality across bioregions and subregions means that results are widely applicable. Further information about how to undertake a BA-specific hazard analysis is found in Section 3.2.2 of this submethodology and in the companion submethodology M11 (as listed in Table 1) for hazard analysis (Ford et al., 2016). Hazard analyses undertaken for individual subregions are available as datasets from product 2.3 (conceptual model of causal pathways).
2.3.2 A quantitative analysis of impacts and risks
There are many different definitions and formulations of risk, with notable differences between application domains. It is common to distinguish the likelihood from the consequence. The AS/NZS ISO 31000:2009 standard on risk management (mandated by the BA methodology) departs from this and considers risk as ‘the effect of uncertainty on objectives’ and seeks to describe what could happen given the uncertainty in external and internal factors and influences, and how the objectives may be affected. Within BAs the objective is the community’s desire to protect the water-dependent assets that they value. The specific objectives related to individual assets are, however, unknown and require careful consideration of a number of non‐scientific matters and value judgments. Therefore the risk evaluation and imposition of risk management strategies that typically occur as part of a broader regulatory process are beyond the scope of BAs; these are roles of proponents and government regulators.
There are many different ways to calculate risk, with qualitative, semi-quantitative and fully quantitative approaches. Within BAs a quantitative or probabilistic approach to risk is adopted. The hazard analysis identifies those risks, and the assessment seeks to understand those risks by assessing the impact (or consequence), the likelihood of that impact, and the uncertainty associated with the likelihood (represented by probabilities, see Section 3.2.4).
In BAs, qualitative descriptors are defined quantitatively. For example, where a high/medium/low scale is used to communicate the results of an assessment, the quantitative cut-offs between the classes are explicit. This protects against different interpretations of these terms by different audiences, and allows for coherent calculation of the uncertainty in the risk predictions (Section 2.3.3.; Lindley, 2006)
Concepts and quantities used are well defined and, where appropriate, measurable, at least conceptually. As an example, abstract concepts such as ‘ecosystem health’ have not been used if they are not defined explicitly in terms of measurable quantities such as, for example, number of species, or biomass. This restriction helps protect against linguistic uncertainty and the ensuing misunderstandings this creates because of the ambiguity of natural language, and means that predictions can be tested against data in the future.
2.3.3 Assessment of regional-scale cumulative impacts
BAs focus on the cumulative impacts of coal resource developments at a regional scale, rather than specifically on individual mines or CSG operations. Coal resource developments in a bioregion or subregion typically comprise a suite of developments, which are distributed across a bioregion or subregion at variable distances from each other and have variable, but often overlapping periods of operation. Thus there is potential for the impacts to accumulate to varying degrees in both space and time.
Regional-scale models allow the assessment to address some of the complexity challenges in a bioregion or subregion, and are used to predict the cumulative hydrological changes and potential impacts of those developments on ecosystems and water-dependent assets from multiple developments over time. The area of potential impact may often be more extensive and extend greater distances downstream of developments than what is predicted from site-scale, single mine models. In some cases the spatial or temporal alignment of certain coal resource developments can allow for attribution of potential effects to individual developments, but that occurs because of that alignment rather than by design.
Results of a BA impact and risk analysis do not replace the need for the detailed site- or project-specific investigations that are currently required under existing state and Commonwealth legislation. The hydrological and ecological systems modelling undertaken for a BA is appropriate for assessing the potential impacts on and risks to water resources and water-dependent assets at the ‘whole-of-basin’ scale, whereas the modelling undertaken by a proponent for an individual coal resource development, as part of an environmental impact assessment, occurs at a much finer scale and makes use of local information to more accurately represent the local situation. Therefore, results from detailed specific coal resource development studies are expected to differ from those from a BA. BA results should not be used to invalidate existing site-specific modelling or impact assessments.
2.3.4 A focus on predictive uncertainty
Probability is used to represent uncertainties in BAs. These probabilities are interpreted as representing an individual’s (for example, an expert’s or analyst’s) degree of belief in an uncertain event given their current knowledge base (Lindley, 2006). This choice naturally accommodates expert opinion, which is an important part of the analysis and ensures that uncertainties are propagated coherently (i.e. in accordance with the standard laws of probability).
The BA methodology (Barrett et al., 2013) defines key aspects of the scope of the analysis and imposes constraints on the uncertainty analysis by specifying that some components of the problem are to be considered constant or fixed at the time an individual expresses their degree of belief about an uncertain event. Nonetheless, the scope of BA poses a considerable challenge, and for processes and activities that are in scope, a choice needs to be made about whether to acknowledge uncertainty or to choose a particular possibility and fix this in the analysis. Practically, this requires the risk analysis to specify which parts of a problem are considered to be uncertain and which parts must be conditioned on (i.e. taken as fixed).
Four examples illustrate the application of these choices:
- BAs do not assess the effects of uncertainty in the CRDP. It is not known exactly what developments will occur and when they will occur as this depends on regulation, market conditions and other socioeconomic or political factors. The assessment is based on a single most likely pathway for coal mining and CSG development in the bioregion or subregion (companion submethodology M04 (as listed in Table 1) for developing a coal resource development pathway (Lewis, 2014)). Multiple development pathways are not generated or evaluated in the BAs due to the increased level of uncertainty in the analysis that this would create.
- Uncertainty in the impacts caused by risks that are currently managed under existing industry standards or regulatory processes, such as the failure of a tailings dam or incomplete well casings, are not considered. Although these types of failure may have socioeconomic or ecological consequences, they are addressed through industry standards or site-based risk management and are not therefore considered as part of the assessment or the uncertainty analysis.
- The impacts due to coal resource development will depend on the climate over the assessment period. For example, a reduction in groundwater, or a discharge of produced water, would have a different impact depending on whether there was a sustained drought or a period of above average rainfall. A pragmatic choice has been made to fix the climate within BAs to a single ‘mid-range’ future climate time series (companion submethodology M06 (as listed in Table 1) for surface water modelling (Viney, 2016)). The BA methodology restricts the scope to impacts due only to coal resource development. The level of agricultural, industrial and urban development is specified as fixed at the baseline extent. The effect of changes in (for example) agricultural practice, and the uncertainty that this creates, has not been incorporated into the assessment because the focus of BA is on the difference between two coal resource development futures.
- BAs focus solely on water-related impacts, and specifically those related to water quantity and availability. Potential water quality hazards are identified through the comprehensive hazard analyses, but the analysis, as determined by the BA scope, is limited to salinity and is only addressed qualitatively.
For those parts of the problem that are considered uncertain, there is central focus on characterising the range of potential hydrological outcomes (i.e. changes to surface water or groundwater) and, where appropriate, the range of potential outcomes in ecologically relevant receptor impact variables, by considering parameter uncertainty as fully as possible in all predictions. For example, groundwater models are run many thousands of times using a wide range of plausible input parameters for many of the critical hydraulic properties, such as the hydraulic conductivity and storage coefficients of all modelled hydrogeological layers. This differs from the traditional deterministic approach used more routinely for surface water and groundwater modelling and is driven by the risk analysis focus of BAs. The quantitative representation of the predictive uncertainty through probability distributions allows BAs to consider the likelihood of impacts or effects of a specified magnitude and underpins the impact and risk analysis. Numerical models are created to represent a simplified conceptual understanding of the system. Where there are sources of uncertainty that those models are unable to incorporate quantitatively their effects, particularly on predictions, are considered qualitatively.
2.3.5 Hydrological predictions for any location in the landscape
The chain of causation depicted in Figure 5 shows that an assessment of the potential impacts and risks to a water-dependent asset requires prediction of the hydrological change for that asset. In order to represent any hydrological or ecological change that asset may experience, it is necessary to make predictions for the full extent of that asset. The extent of assets is large, and therefore effective predictions need to be made at any location in the bioregion or subregion.
There are, of course, practical limits and constraints to this. Hydrological models have their own resolutions, whether that be the node-link model resolutions of surface water models or the possibly variable sizes of grid cells in groundwater models as described in companion submethodology M06 (as listed in Table 1) for surface water modelling (Viney, 2016) and companion submethodology M07 for groundwater modelling (Crosbie et al., 2016). However, to reliably assess potential impacts, outputs from those hydrological models need to be interpolated to locations that are relevant to water-dependent assets.
The locations where predictions are required are divided into square grids of assessment units. The size of the grid cell used is flexible but in practical implementation ranges between 500 x 500 m in the Gloucester subregion and 1500 x 1500 m in the Maranoa-Balonne-Condamine subregion, with most subregions at 1000 x 1000 m resolution. The choice of resolution is primarily driven by the groundwater model resolution. Impacts on and risks to particular assets or landscapes classes may be assessed by aggregating predictions across the assessment units that pertain to that asset or landscape class. The assessment units are tagged with other information, such as details of the landscape class and relevant hydrological response variables and receptor impact variables.
The assessment unit supersedes the use of receptors as points in the landscape where predictions are required and water-related impacts assessed (as originally specified in the BA methodology and companion submethodology M03 (as listed in Table 1) for assigning receptors to water-dependent assets (O’Grady et al., 2016). Conceptually, assessment units contain an infinite number of receptors and hence can be used as a multi-purpose spatial device to map any number of potential impacts for a range of receptors identified within water-dependent assets and/or landscape classes.
2.3.6 Decomposition of the predictions into conditionally independent components
Figure 5 outlines the logical basis of the analysis. Conditional on coal resource development, hydrological predictions are made with associated uncertainty. Conditional on a given hydrological change (and the scope and analysis restrictions described in Section 2.3.3), a receptor impact model predicts the possible ecological outcomes. Thus, uncertain ecological or economic impacts can be estimated from uncertain hydrological impacts.
Decomposing the workflow and associated predictions into components ensures that the assessment is, where possible, modular. Changes or updates to one component should not necessarily trigger changes to all other components. This means that future updates or iterations to a BA do not have to revisit each component of work to the same level and intensity as done during the initial BA. For example, if the CRDP was to change, adjustments to some components of work may be needed (e.g. incorporating new coal resource developments in the groundwater model) but may not affect many other components (e.g. the water-dependent asset register). If an improved surface water model becomes available, the modelled hydrological changes could be updated as part of the assessment workflow in a future BA. Although there is effort and expertise required in any update, the modular nature of the assessment means that effort is relatively much reduced.
2.3.7 Landscape classification
A bioregion or subregion is a complex landscape with a wide range of integrated human and ecological systems. Because of this complexity a direct analysis of each and every point in the landscape across the bioregions and subregions is not currently possible. Abstraction and a systems-level classification help manage the challenges of the dimensionality of the task.
In each bioregion and subregion, a set of landscape classes is defined that are similar in their physical, biological and hydrological characteristics (refer to companion submethodology M05 (as listed in Table 1) for developing a conceptual model of causal pathways (Henderson et al., 2016) for additional information on landscape classes). This reduces the complexity of the analysis task in each bioregion or subregion and is appropriate for a regional-scale assessment. Landscape classes provide a structure that helps the analysis to focus on the key processes, functions and interactions that determine how ecological systems respond to changes in hydrological variables.
The Assessment team chooses the method for landscape classification, but wherever possible they build on existing, well-accepted classifications such as the Australian National Aquatic Ecosystem (ANAE). Substantial previous time and effort has gone into constructing and defining systems of landscape classification relevant to the Bioregional Assessment Programme. The Programme has sought to understand the limitations and relevance of potential classification systems, and thus has developed and justified a consensus choice for the classification or combination of classifications that best matches the demands of a given bioregion or subregion. The reality is that no one existing classification is likely to fully satisfy the needs of the analysis given that the interests of BAs fall across both ecological systems and human systems (including agricultural production systems, industrial and urban uses). The choice is guided by the overall objective of reducing the complexity of the analysis task, which can be achieved by choosing a classification where the individual classes are as homogeneous as possible in their response to the water-related impacts of the coal resource development. There is a trade-off between adding new classes to achieve homogeneity and increasing the specificity of the resulting analysis. If an assessment was undertaken at a different spatial scale, an alternative classification may be appropriate in getting that balance right.
The landscape classification also allows the effort to be concentrated on those landscape classes that are water dependent. An additional consideration is that in large bioregions or subregions, water-dependent landscape classes may not be near coal resource developments so are very unlikely to be impacted. The Assessment team should use the hazard analysis and preliminary hydrological modelling to analyse the spatial extent (or footprint) of important CSG- and coal mine-related hazards, and exclude from detailed analysis those landscape classes with negligible potential impact.
2.3.8 Two assessment time points
The receptor impact models are constructed to predict receptor impact variables (representing ecological changes) at particular points in time. The potential impact can depend strongly on the time of that assessment, and may be complicated by the (potentially) long lags that can occur in groundwater systems and between the hydrological change and ecological response.
Predictions are restricted to two time points given that the significant uncertainties about the dynamics of these ecosystems translates into large uncertainties in the experts’ predictions. This restricted analysis is simpler to perform.
In BAs, ecosystem impacts are considered for two time points: 2042 and 2102. The reference year of assessment is 2012. Broader hydrological changes are also considered as maximum impacts across the full 90-year time series from 2013 to 2102.
The time point 2042 for ecosystem impacts is chosen based on a number of considerations. First, the nature of the CRDP means that the features of the identified developments may quickly change over time (e.g. the mine design and scheduling originally proposed in the development application or environmental impact statement may be modified), so short-term analyses are important. In addition, the broader community will be naturally interested in the changes that they will experience in their lifetimes. This suggests choosing a time point in the relatively near future and at the height of coal resource development.
While surface water hydrological regimes may return to something close to their pre-development state in the short-to-medium term after site rehabilitation and closure, groundwater impacts may continue to occur over the longer term and ecological systems may not respond as quickly. The time point 2102 is chosen to represent the enduring impacts of these developments to the landscape.
2.3.9 Use of experts where empirical data are limited
Expert elicitation is central to a BA. It supports the choice of some parameter ranges for the numerical hydrological models. It underpins the receptor impact modelling by summarising the range of the potential ecosystem response for a given change in hydrology.
In many cases limited data are available to make formal inference from. Because of this, expert interpretation and opinion will be needed to form a coherent assessment. The use of experts requires considerable care and effort. Poorly staged elicitation approaches can lead to frustration and disengagement of key experts, leading to poor quality and potentially biased data. Facilitation must ensure that the questions are clearly defined and interpretable by the experts. Wide variation in opinions between experts means that the experts must be carefully chosen in consultation with the client. Motivation of the experts is also essential and again the client may be able to assist and increase participation by key experts in the BA process.
It is important to develop a tractable elicitation scenario that is not too difficult or complex for general experts, due to the large number of bioregions or subregions and landscape classes combined with multiple timescales, receptor impact variables and hydrological response variables to consider within each receptor impact model. This is achieved by careful design of the elicitation process, drawing on the principles of optimal experimental design (companion submethodology M08 (as listed in Table 1) for receptor impact modelling (Hosack et al., 2018a)).
2.3.10 Qualitative mathematical modelling to estimate direct and indirect impacts and choose key variables
Qualitative mathematical modelling is described in detail in companion submethodology M08 (as listed in Table 1) for receptor impact modelling (Hosack et al., 2018a). It has several key roles in a BA: (i) it provides a graphical conceptualisation of the landscape ecosystem, identifies the critical hydrological processes that support the ecosystem’s components and processes, and identifies how these may change due to coal resource development; (ii) it enables the Programme to predict the changes due to direct and indirect impacts on the ecosystem; and (iii) it provides a transparent mechanism for selecting hydrological response variables and receptor impact variables to be used in receptor impact models.
An example of a signed digraph (SDG) output from the qualitative mathematical modelling for the ‘Perennial – gravel/cobble streams’ landscape class in the Gloucester subregion is presented in Figure 6 to provide context for a description of the two roles that follows.
Example only; do not use for analysis. This is an early draft of a figure published in companion product 2.7 for the Gloucester subregion (Hosack et al., 2018b). See Hosack et al. (2018b) for full explanation. Variables are: bank stability (BS), fine particulate organic matter (FPOM), fine sediments (FS), flow regimes (FR1, FR2 and FR3), groundwater (GW), high-flow macroinvertebrates (HF MI), herbaceous vegetation (includes aquatic macrophytes) (HV), large woody debris (LWD), platypus (Platy), primary production (PP), precipitation (Ppt), predators (Pred 1 and Pred 2), regent honeyeater (RHE), seedlings (Seedl), slow-flow macroinvertebrates (SF MI), stuttering frogs (SF), still-water macroinvertebrates (SW MI), upstream recruitment (Ur), wading and diving birds (W&DB); woody riparian vegetation (WRV).
2.3.10.1 Direct and indirect impacts
The BA methodology (Barrett et al., 2013) requires ‘explicit assessment of the potential direct, indirect and cumulative impacts of CSG and coal mining development on water resources’ together with an analysis of the associated uncertainties, but does not provide guidance on how this will be achieved. The BA methodology defines direct and indirect impacts, respectively, as:
- ‘… those associated with CSG and coal mining developments that impact on natural resources without intervening agents or pathways’
- ‘… those impacts on receptors (within water-dependent assets) that are produced as a result of a pathway of cause and effect. This causal pathway may be simple or complex. Sometimes indirect impacts are referred to as second- or third-level impacts, or secondary impacts (Walker and Johnston, 1999)’.
To operationalise these definitions, direct and indirect impacts need to be identified in an unambiguous fashion in a stressor conceptual model – that is, a conceptual model that identifies how stressors (here, changes to hydrological processes) interact with components and processes of the ecosystem.
Direct and indirect impacts may be distinguished via qualitative mathematical modelling by presenting the stressor conceptual model for an ecosystem (landscape class) as a signed digraph. From the graph structure it is possible to examine the causal pathways that link hydrological response variable to the receptor impact variable. Pathways with just one link in the SDG are formally direct impacts on the receptor impact variable. Pathways with two or more links, which thereby involve other system variables, are formally indirect impacts.
The principal concern in BAs is with press perturbations – that is, changes in hydrological response variables that are sustained for a relatively long time period, for example, over much larger time frames (many decades) than the generation times of the potential receptor impact variables (days to decades) The sustained nature of this type of perturbation, in contrast to pulse perturbations, provides time for the knock-on effects to be felt throughout the entire system.
By representing conceptual models of landscape classes as SDGs, it is possible to make qualitative predictions of impacts to variables (i.e. direction but not magnitude of change) from sustained hydrological changes. This analysis enables comparison of the predicted direction of change from the qualitative mathematical analysis with the quantitative analysis of the receptor impact variable.
2.3.10.2 Choice of hydrological response variables and receptor impact variables
Qualitative mathematical modelling supports two of the most important choices during the receptor impact modelling: the choice of hydrological response variables and receptor impact variables.
There are typically tens to hundreds of potential hydrological response variables that can be modelled using the groundwater and surface water models, but the receptor impact modelling must by necessity be limited to using just a few per landscape class to ensure that the elicitation requirements of the subsequent receptor impact models are reasonable and achievable. The choice of hydrological response variables to include in the receptor impact models should be guided by the results of the hazard analysis, and the constraints and restrictions on the scope of the analysis (Section 2.3.3). As BAs are primarily concerned with press perturbations, the characteristics of extent, magnitude, duration and rate of impact should also influence the choice of hydrological response variables.
There are also typically hundreds to thousands of potential receptor impact variables, which are indicators of ecosystem condition, across the assets of a bioregion or subregion. This choice is circumscribed to some extent by the definition of landscape classes, but still within the qualitative mathematical models and conceptual models of these landscape classes tens of potential receptor impact variables may be anticipated, when by necessity the limitation is for no more than a few per landscape class given workshop logistics and constraints with expert availability. The complexities of the potential direct and indirect effects associated with press perturbations suggest a priori that receptor impact variables at the base of complex food webs are less likely to be involved in complex indirect impact pathways, thereby making the elicitation task more tractable and focusing the analysis on a key underpinning component of the system. The generation time of the receptor impact variables, in the context of press versus pulse perturbations, should also be considered and should also influence this choice.
The choice of impact variables also needs to be framed by the expected audience, which includes the primary audience of the Independent Expert Committee on Coal Seam Gas and Large Coal Mining, regulators, and industry proponents, but expands to wider range of interests for the community. There will be experts about particular species and experts about particular locations. There will be experts about particular agricultural or ecological systems and experts about particular taxa. Members of the general public will each have their own beliefs and understandings of these systems, and an associated set of values. The Programme needs to be conscious of the expectations of this community, and the natural tension that arises between ensuring that the risk analysis is achievable (with the current operational constraints) but at the same time relevant to as much of the community as possible. This can be achieved, for example, by choosing receptor impact variables that simplify the elicitation task and speak to broad sections of the community, and by using the narrative in the analysis to broaden the assessment to other more specific sections of the community. For example, basal vegetation variables can speak to diverse segments of the expected audience when interpreted, for instance, in terms of forest cover. Companion submethodology M08 (as listed in Table 1) for receptor impact modelling (Hosack et al., 2018a) describes the specific criteria used to guide the selection of receptior impact variables in more detail. These include:
- Is the response variable directly affected by changes in hydrology? These variables typically have a lower trophic level, and focusing on direct (signed digraph arcs of length one) impacts helps alleviate the elicitation burden imposed on experts during the construction of the receptor impact models.
- Is its status important in maintaining other parts of the landscape class? Variables (or nodes) within the qualitative model that other components of that ecosystem or landscape class depend on will speak more broadly to potential impacts. Again, these types of variables will typically have a lower or mid-trophic level.
- Is it something that the available expertise can provide an opinion on? There is a need to be pragmatic and make a choice of receptor impact variable that plays to the capabilities and knowledge base of the experts that are available at the time the receptor impact models are created.
- Is it something that is potentially measurable? This is essential for (in)validation of the predicted impacts and in the subsequent design of monitoring strategies that close the risk analysis loop by testing, comparing its predictions with observations.
- Will the community understand and accept the relevance and credibility of the receptor impact variables for a given landscape class? This reflects the communication value of the receptor impact variable.
2.3.11 Automation of the analysis
The task of predicting impacts and risks is substantial. The bioregions are large; the water-dependent assets are numerous, extensive and overlapping; and the many potential hydrological and ecological changes are relevant and need to be summarised in various ways.
Therefore, a systematic automated approach is used to assess the impacts on and risks to landscape classes and water-dependent assets. This requires a common spatial resolution of the assessment unit across a given bioregion or subregion (typically 1000 x 1000 m, based primarily on the underlying resolution of the groundwater modelling) so that results at these assessment units can be aggregated to different scales: regional, landscape class or individual asset. A key aspect of this approach is the translation from a spatial database representation to a relational database representation using the assessment units as the construct that holds and transfers the information. More details are outlined in Appendix A.
This automation ensures consistency and the ability to meet the key transparency requirement of the Programme.
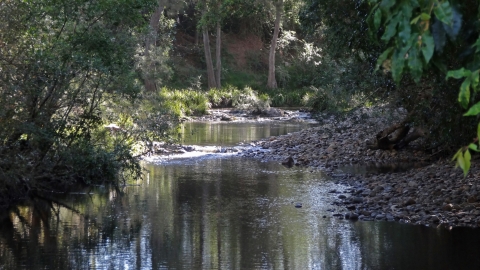
METHODOLOGY FINALISATION DATE
- 1 Introduction
- 2 Design choices for the impact and risk analysis
- 3 High-level logic and workflow
- 4 Impact and risk predictions for assets and landscape classes
- 5 Reporting and communicating impacts and risks
- 6 Building on the impact and risk analysis
- Appendix A Methods for structuring and processing data for bioregional assessment impact and risk analysis purposes
- References
- Datasets
- Citation
- Acknowledgements
- Contributors to the Technical Programme
- About this submethodology