2.5.2.1.1 Model Node 53 – Belyando River surface water balance
Surface water balances at surface water model node 53 (Figure 3 of Section 2.5.1) on the Belyando River are provided in Table 3 for time period 2013 to 2042, Table 4 for 2043 to 2072 and Table 5 for 2073 to 2102. The surface water balance applies to an area of 35,326 km2 on the Belyando river basin, which represents a proportion of the river basin upstream of node 53. Rainfall inputs over the three time periods reflect changes due to a varying climate signal as described in Section 2.5.1.1.
Each table presents the difference in results under the CRDP and the baseline. The difference in results is attributable to only the additional coal resource developments (i.e. the seven coal projects in the CRDP that can be modelled), as the climate signal is identical for baseline and CRDP. The differences between corresponding values over the three reporting periods encompass both the effects of climate and the coal resource development.
The climate change signal exhibits as an overall decrease in mean annual rainfall. Any rainfall that does occur is also increasingly concentrated into the summer rainfall period. The decrease in mean annual rainfall is about 2% and 4% for the period 2043 to 2072 and 2073 to 2102 respectively compared to the 2013 to 2042 period. Surface water outflow, however, shows an increasing trend of approximately 1% and 2% for the same time period which is equivalent to approximately 21 and 42 GL/year respectively. The explanation for this contrasting behaviour between mean annual rainfall and streamflow is that the increase in summer rainfall coincides with the season when most of the streamflow occurs (see companion product 1.1 for the Galilee subregion (Evans et al., 2014)).
The major difference in the surface water balance between the baseline and the seven coal projects modelled from the CRDP is in the surface water outflow. This has a maximum difference of 13 GL/year in the 2013 to 2042 period (Table 3), with decreasing differences in the subsequent two 30-year periods (Table 4, Table 5). Most of this difference can be attributed to interception of surface runoff rather than groundwater extraction because for all three periods of time the changes in baseflow are very small. The decrease in surface water outflow is reflected in an increase in the water balance losses term. Most of the water lost is from evapotranspiration, although leakage of water from open-cut mines and mine water storages may account for a small component. The decrease in surface water outflow under the CRDP is less than 1% of the baseline surface water outflow and within the uncertainty of the predicted surface water outflow (as shown by the range of surface water outflow estimates at the 10th, 50th and 90th percentiles for the three respective reporting periods in Table 3 (2013 to 2042), Table 4 (2043 to 2072) and Table 5 (2073 to 2102)).
Table 3 Mean annual surface water balance at model node 53 on the Belyando River for 2013 to 2042 in the Galilee subregion
aFor some (but not all) terms, three numbers are provided. The first number is the median, and the 10th and 90th percentile numbers follow in brackets.
baseline = baseline coal resource development, ET = evapotranspiration
The coal seam gas and coal projects in the coal resource development pathway (CRDP) are the sum of those in the baseline and in the additional coal resource development (ACRD). Because there are no coal resource developments in the baseline for the Galilee subregion, the CRDP includes only the proposed coal seam gas and coal projects in the ACRD. Results are presented for seven coal projects that had sufficient data to have been included in numerical hydrological modelling.
Data: Bioregional Assessment Programme (Dataset 1, Dataset 2)
Table 4 Mean annual surface water balance at model node 53 on the Belyando River for 2043 to 2072 in the Galilee subregion
aFor some (but not all) terms, three numbers are provided. The first number is the median, and the 10th and 90th percentile numbers follow in brackets.
Baseline = baseline coal resource development, ET = evapotranspiration
The coal seam gas and coal projects in the coal development resource pathway (CRDP) are the sum of those in the baseline and in the additional coal resource development (ACRD). Because there are no coal resource developments in the baseline for the Galilee subregion, the CRDP includes only the proposed coal seam gas and coal projects in the ACRD. Results are presented for seven coal projects that had sufficient data to have been included in numerical hydrological modelling.
Data: Bioregional Assessment Programme (Dataset 1, Dataset 2)
Table 5 Mean annual surface water balance at model node 53 on the Belyando River for 2073 to 2102 in the Galilee subregion
aFor some (but not all) terms, three numbers are provided. The first number is the median, and the 10th and 90th percentile numbers follow in brackets.
baseline = baseline coal resource development, ET = evapotranspiration
The coal seam gas and coal projects in the coal resource development pathway (CRDP) are the sum of those in the baseline and in the additional coal resource development (ACRD). Because there are no coal resource developments in the baseline for the Galilee subregion, the CRDP includes only the proposed coal seam gas and coal projects in the ACRD. Results are presented for seven coal mines that had sufficient data to have been included in numerical hydrological modelling.
Data: Bioregional Assessment Programme (Dataset 1, Dataset 2)
2.5.2.1.2 Conceptual groundwater balance for the portion of the Burdekin river basin that lies within the Galilee subregion
As outlined in Section 2.5.1.1.2, the analytic element groundwater model was not designed to estimate historical and future water balances. Thus, an alternative conceptual groundwater balance was developed in order to compare baseline conditions (no coal resource development) with a period 30 years in the future that assumes the full development of the coal projects in the CRDP for which there are sufficient data for modelling. Furthermore, the conceptual groundwater balance only focuses on the main components of the groundwater balance, including: recharge, discharge, storage, and changes to storage through groundwater use. While this represents a relatively simple approach by aggregating the various components of the groundwater balance, it is considered fit for purpose for this BA as it will highlight the potential for changes to occur to the water balance components through cumulative groundwater pumping for mining operations.
The conceptual groundwater balance is limited to seven coal projects (Hyde Park, Carmichael, Alpha, Kevin’s Corner, China First, China Stone, and South Galilee) out of a total 17 coal or coal seam gas developments in the CRDP due to data availability. With the exception of Hyde Park, all of the mine projects listed previously have EIS documentation available. However, due to the variability of existing data it is not possible to model the groundwater balance for the same three future time periods as was the case for the surface water balance (see Section 2.5.1.1.2).
The conceptual groundwater balance (Table 6) focuses on the portion of the Galilee subregion (Figure 4) that falls within the Burdekin river basin. This area was selected for the groundwater balance calculation because the seven coal projects in the CRDP that were modelled are located in the Burdekin river basin (see Section 2.3.4 in companion product 2.3 for the Galilee subregion (Evans et al., 2018a)). It also encompasses the river basin area covered by the surface water balance (Section 2.5.2.1.1) and the area where groundwater drawdown is predicted to occur (for further detail see Section 2.6.2.8 in companion product 2.6.2 for the Galilee subregion (Peeters et al., 2018)).
The groundwater balance consists of inputs (rainfall and surface water recharge) and outputs (bore water use, mine dewatering, discharge to rivers and springs, diffuse evapotranspiration (ET) and groundwater flow out of the modelling area). The conceptual groundwater balance (Table 6 and Figure 5) assumes that prior to drilling of groundwater bores the groundwater system was in a steady-state condition with inputs equal outputs. Thus prior to coal resource development in the region:
Rainfall recharge + surface water recharge = Evapotranspiration + baseflow + spring discharge + groundwater flow out of modelling area.
As outlined in Section 2.5.1, for the purposes of the conceptual water balance, baseflow is assumed to be derived from fully saturated groundwater discharge from an aquifer to surface drainage. This may provide a theoretical upper estimate of the potential fluxes of groundwater that may occur between river reach and an underlying aquifer.
Current groundwater bore use in the modelling region began in the late 1940s and in 2012 it represented approximately 4% of groundwater recharge. Because of the low water use relative to groundwater recharge and the relatively short period of use, the conceptual groundwater balance assumes that at the regional scale, groundwater is considered to be in a quasi-steady-state equilibrium. A groundwater system not in equilibrium can occur where natural discharge exceeds current recharge. This can be the case in large aquifer systems where groundwater has a long residence time or in aquifers with complex flow pathways. This can have implications for the amount of groundwater that is potentially available for abstraction. This assumption can be tested through better understanding of groundwater flow pathways and the use of environmental isotopic tracers.
As can be seen in Table 6, “under the baseline” natural inputs (recharge) are equal to natural outputs (springs, evapotranspiration losses and surface water discharge). The negative change in storage is equal to bore groundwater use.
In the modelled CRDP, natural inputs are again equal to natural outputs with the negative change in storage being due to bore groundwater use and mine water use. In reality, the relatively high mining water use is likely to change (the assumed) quasi-steady-state conditions, which will result in a decrease in groundwater discharge to ET and groundwater flow outside of the modelling area. However, the conceptual groundwater balance is not capable of predicting what these changes may be.
In addition to the combined groundwater balance (Table 6), a separate water balance is also available for the eight major hydrostratigraphic units identified in Section 2.1.3 within the groundwater modelling region (alluvium, Cadna-owie – Hooray aquifer, Hutton Sandstone aquifer, Moolayember Formation aquitard, Clematis Group aquifer, Rewan Group aquitard, upper Permian coal measures partial aquifer, and Joe Joe Group partial aquifer) in Bioregional Assessment Programme (Dataset 1).
Only coal resource developments included in the additional coal resource development are show on the figure
Geological units that underlie the alluvial cover are outlined on the map. Alluvium is not shown.
Data: Bioregional Assessment Programme (Dataset 3, Dataset 4)
Geological units that underlie the alluvial cover are outlined on the map. Alluvium is not shown.
Data: Bioregional Assessment Programme (Dataset 3)
Table 6 contains the major components of the conceptual groundwater balance. The detailed groundwater balance can be found in Bioregional Assessment Programme (Dataset 1), which also includes data source, currency, a measure of data accuracy and the method used to derive the data. The volumes in Table 6 do not take into account changes to recharge due to future climate variations.
Table 6 Mean annual groundwater balance for the Belyando river basin part of the Galilee Basin at baseline (2012) and during mining (2042)
baseline = baseline coal resource development, ET = evapotranspiration
Change in groundwater storage = inflow components – outflow components
Difference = Baseline – Changes predicted under the modelled coal resource development pathway
Changes predicted under the coal resource development pathway relate only to the seven coal projects for which sufficient data were available for modelling
Table 6 is a conceptualised water balance model. For instance, results from more sophisticated modelling approaches (e.g. Peeters et al. (2018) and GHD Pty Ltd (2013a)) predict varying degrees of impact to the springs and river discharge during mining. No change to spring flow or groundwater outflow was considered as part of the conceptual water balance and baseflow.
Data: Bioregional Assessment Programme (Dataset 5)
In 2012, the change to groundwater storage was due to groundwater extraction by groundwater pumping but bores not associated with coal mine development. The projected changes to groundwater storage in 2042 are primarily due to dewatering due to coal production from the seven coal mining projects included in the modelled CRDP.
The following sections provide further detail on individual components of the conceptual groundwater balance as outlined in Table 6.
Groundwater recharge
Mean groundwater recharge for the Burdekin river basin portion of the Galilee subregion was calculated using the chloride mass balance method as described in Section 2.1.3.2.5 of companion product 2.1-2.2 for the Galilee subregion (Evans et al., 2018b). As such, no distinction is made between rainfall recharge and stream recharge. Recharge represents the main input to the groundwater balance. Unlike the approach taken for the surface water balance (Section 2.5.1.1), no allowance was included for a projected declining rainfall trend.
For the conceptual groundwater model, the groundwater recharge estimates produced by Evans et al. (2018b) were clipped to the conceptual groundwater model boundary. The results (groundwater recharge in mm/year) were multiplied by the area of each aquifer and then converted to ML/year. Total groundwater recharge for the portion of the Belyando river basin that occurs in the Galilee subregion is 72,863 ML/year (Table 7 and Figure 5). Recharge estimates in Table 7 are independent volumes representing recharge from rainfall only. Recharge was assumed not to vary due to changes in climate.
Table 7 Groundwater recharge
Data: Bioregional Assessment Programme (Dataset 5)
Bore groundwater use
Bore groundwater use is an outflow component from the groundwater balance. As of 2012 there are 353 groundwater bores within the groundwater balance area of which 119 bores had aquifer information. The remaining bores were proportionally assigned to aquifers based on the known aquifer distribution (Table 8 and Figure 5). The existing groundwater bores are used for town water supply, stock and domestic or agricultural purposes.
The vast majority of groundwater bores (344) have no direct groundwater use data and were therefore allocated a nominal value consistent with stock and domestic use of 5 ML/year. Companion product 1.5 for the Galilee subregion (Evans et al., 2015) provides further detail on water allocations and accounting in the Galilee subregion.
Consistent with the relatively low groundwater use (3.6% of groundwater recharge), there is also relatively low growth in the number of groundwater bores. In the decade from 2002 to 2012, an additional four bores were drilled in the region or approximately 1.3% growth per decade. This equates to an increase of approximately 96 ML/year in 2042 or an additional 19 bores. Despite the projected increase in consumption, overall groundwater use based on these figures would still be below 4% of groundwater recharge.
Table 8 Current groundwater extraction as of 2012
Data: Bioregional Assessment Programme (Dataset 5)
Mine groundwater use
Groundwater inflows to coal mines and dewatering around mine areas (either open-cut or underground) represent an output from the groundwater balance. It will only occur once mining development commences. As outlined in Table 6, at the time of the baseline estimate (2012) there was no water associated with this component of the groundwater balance as there were no coal projects in operation. This is still the case at the time of writing (March 2016). Note that all groundwater pumped as part of mine development will be utilised at mine site as part of their water management strategies (see Section 2.1.6 in Evans et al. (2018b) and Section 2.3.4.2 in Evans et al. (2018a)).
Table 9 outlines the mine groundwater usage for the seven coal projects included in the modelled CRDP 30 years after development commences. It is estimated that groundwater inflows to mine areas may be the equivalent of up to 66% of the calculated groundwater recharge volume (Table 6).
None of the mine water balances outlined in EIS documentation reference the same time period. As an example, Table 10 outlines the original dewatering figures as reported for the six projects for which an EIS has been submitted to regulatory authorities for consideration. As of April 2016, an EIS had not been submitted for Hyde Park project.
Table 9 Estimated groundwater use by proposed mine derived from groundwater inflows to mine areas
Mine |
Mine water use at 2042 from product 2.6.2 (ML/y) |
---|---|
Hyde Park |
1,095 |
China Stone |
4,928 |
Carmichael |
5,001 |
Kevin’s Corner |
3,176 |
Alpha |
1,935 |
China First |
22,995 |
South Galilee |
9,016 |
Total |
48,146 |
Data: Peeters et al. (2018), Bioregional Assessment Programme (Dataset 5)
Table 10 Projected mine dewatering volumes (ML/y) throughout production schedule (as outlined in EIS water balance reports)
na = not applicable, NA = data not available (as in the case of Hyde Park).
Data: Evans et al. (2018b), Bioregional Assessment Programme (Dataset 6)
Another water balance related issue, which is beyond the scope of this product, are proposals to obtain water from sources external to the mine development for the six coal mining projects for which an EIS has been submitted. In the EIS documentation, this is termed ‘external water’, which represents water required in addition to that sourced from mine dewatering or rainfall collected on-site.
Estimated volumes and proposed sources for external water requirements are outlined in Table 11. Data presented are sourced from the water balance reports included as part of EIS documentation. In some cases it is not clear whether the external water will be sourced from groundwater or surface water resources, nor if the water will be sourced from either within the Galilee subregion or from elsewhere. Total cumulative external water use for all coal projects could be in the order of an additional 37,657 to 39,320 ML/year (see also Table 11 for further detail).
As of April 2016, the source of external water for the six coal mining projects had yet to be finalised. The cumulative volumes of external water required are substantial, and may represent an increase of approximately 80% over the water that may be obtained through mine dewatering. It should be noted though that this represents the maximum volume that may be required. For instance, if in any given year there was more water available on-site from increased groundwater inflows or wet weather, then it is likely that during those conditions that less external water would be required by mine projects.
Table 11 Proposed external water requirement for mine projects (as outlined in environmental impact statements)
Project |
Proposed external water supply amount (ML/y) |
Expected life of mine (years) |
Total LOM requirement (max) (ML) |
Proposed water sources |
Reference |
---|---|---|---|---|---|
China Stone |
12,500 |
50 |
625,000 |
From water harvest schemes, from Cape River or Belyando Suttor river system |
Chapter 13, p. 25 |
Carmichael |
12,500 |
56 |
700,000 |
Flood water harvester on the Belyando River |
GHD (2013b) Table 3-1, p. 19, 21 and 12 |
Kevin’s Corner |
2,300–2,952 |
30 |
88,560 |
Unnamed third party supplier |
URS (2012) p. 75 |
Alpha |
7,537–8,236 |
30 |
247,080 |
Not stated. 'Out of scope for mine technical reports' |
Parsons Brinckerhoff (2011) Table 5-11, p. 60 |
China First |
2,500 |
25 |
62,500 |
Burdekin River |
|
South Galilee |
320–632 |
32 |
20,224 |
‘The external water source is not known and the other external infrastructure solutions require development in conjunction with other proponents of the South Galilee Basin’ |
AMCI (2014) p. 33, Section 1.7 Scheduling constraints |
Total |
37,657–39,320 |
1,743,364 |
LOM = life of mine
The reference column outlines the source document for the external water volumes presented in the column ‘Proposed external water supply amount’
Data: Bioregional Assessment Programme (Dataset 6)
Springs discharge
Springs represent an outflow component of the groundwater balance. Table 12 and Figure 5 lists the spring complexes included in along with any available estimates of groundwater discharge.
Table 12 Groundwater springs and estimated water use
NA = Not Available as of February 2016
Data: Bioregional Assessment Programme (Dataset 5)
Other groundwater components (evapotranspiration and groundwater flow into water balance area)
The largest components of natural outflow within the groundwater balance model are evapotranspiration (~26%) and groundwater flow out of the modelling area (~57%).
Due to the lack of information, groundwater flow eastwards towards the margin of the subregion is assumed to discharge as: baseflow to rivers, springs discharge, or as evapotranspiration. Groundwater flow westwards is assumed to flow out of the groundwater balance modelling domain area into other parts of the Galilee Basin.
The volume of groundwater associated with losses as ET or groundwater flow out of the groundwater balance domain are based on the need to balance groundwater recharge as shown in Section 2.5.2.1.2.
The groundwater used by the coal projects included in the modelled CRDP will change quasi-equilibrium conditions resulting in a decrease in both ET and groundwater flow out of the modelling area. However, the conceptual groundwater balance is not capable of making this calculation. Therefore, ET and groundwater flow out of the modelling area are shown as unchanged in the future development pathway.
Evapotranspiration
A significant, albeit difficult to calculate, component of the groundwater balance is diffuse groundwater evapotranspiration (ET). Typically this occurs via transpiration from deep-rooted vegetation and/or direct evaporation of shallow groundwater. Evapotranspiration was not calculated using a specific method; rather it was assumed in the discharge component of the water balance equation that some residual loss (i.e. a loss not attributable to bore discharge, spring discharge or baseflow) is assumed to be due to evapotranspiration (Table 13). The evapotranspiration calculation did not take into account the effects of changes in vegetation cover or type across the conceptual water balance model area.
Table 13 Groundwater discharge as evapotranspiration
Data: Bioregional Assessment Programme (Dataset 5)
Groundwater outflows from the water balance model area
For the baseline (2012) water balance model to be in equilibrium, the overall groundwater inflow volume must equal the groundwater outflow volume (see Table 6). Table 14 details the amount of outflow required to the equation. These volumes may be flowing out of the water balance model area. These numbers could be a measure of how much the model may have underestimated one (or all) of the other outflow components. Alternatively, the recharge (inflow) component is overestimated. This represents a significant unknown for the water balance, in particular for the Clematis Group aquifer and the upper Permian coal measures partial aquifer. As outlined in Section 2.5.2.2, more work is required to refine the estimates for the various water balance components.
The conceptual hydrogeological understanding presented in Section 2.3.2 in companion product 2.3 for the Galilee subregion (Evans et al., 2018a) provides evidence that a major groundwater divide exists in the Galilee Basin groundwater systems, and that this groundwater divide is situated to the west of the Burdekin river basin margin. This groundwater divide does not extend along the whole of the western Burdekin river basin margin. Areas exist where groundwater has potential to flow westwards away from recharges areas into the Galilee basin. These areas occur along the Burdekin river basin margin, south of Alpha (Figure 5).
Only small portions of Eromanga Basin aquifer lie within the Burdekin river basin. For the geological Eromanga Basin, the Burdekin river basin boundary forms a localised groundwater divide within unconfined Eromanga Basin aquifers, directing flow east and west of the topographic divide. Groundwater flow in the alluvium is strongly controlled by local topography and so the Burdekin river boundary would coincide with a groundwater divide in the alluvium; therefore, no inflow occurs from outside the river basin boundary. Table 14 presents an estimate of groundwater flow that occurs into the Burdekin river basin.
Table 14 Groundwater outflow component from the portion of the Burdekin river basin that lies within the Galilee subregion
na= not applicable
These numbers are used in the 2012 water balance model to balance the outflow component of the water balance equation against the inflow component. Total outflows is the 2012 water balance should equal groundwater inflows + bore discharge
Data: Bioregional Assessment Programme (Dataset 5)
Discharge to surface water
According to the conceptual groundwater balance model (Bioregional Assessment Programme, Dataset 1), total groundwater discharge (Table 15) to the Belyando and Carmichael rivers is 9,045 ML/year.
Table 15 Groundwater discharge to river
River |
Aquifer discharging to river baseflow |
Estimate of groundwater discharge (ML/y) |
Reference |
---|---|---|---|
Belyando River |
Alluvium |
7,950 |
|
Carmichael River |
Clematis Group/alluvium |
1,095 |
|
Total |
|
9,045 |
|
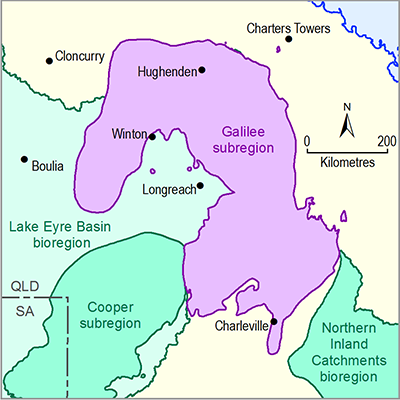